Hollow-core fiber is a novel type of optical fiber. Unlike traditional fibers, which contain a core made of silica glass (primarily composed of silicon dioxide), hollow-core fibers are essentially “empty”—containing only air, inert gas, or vacuum.
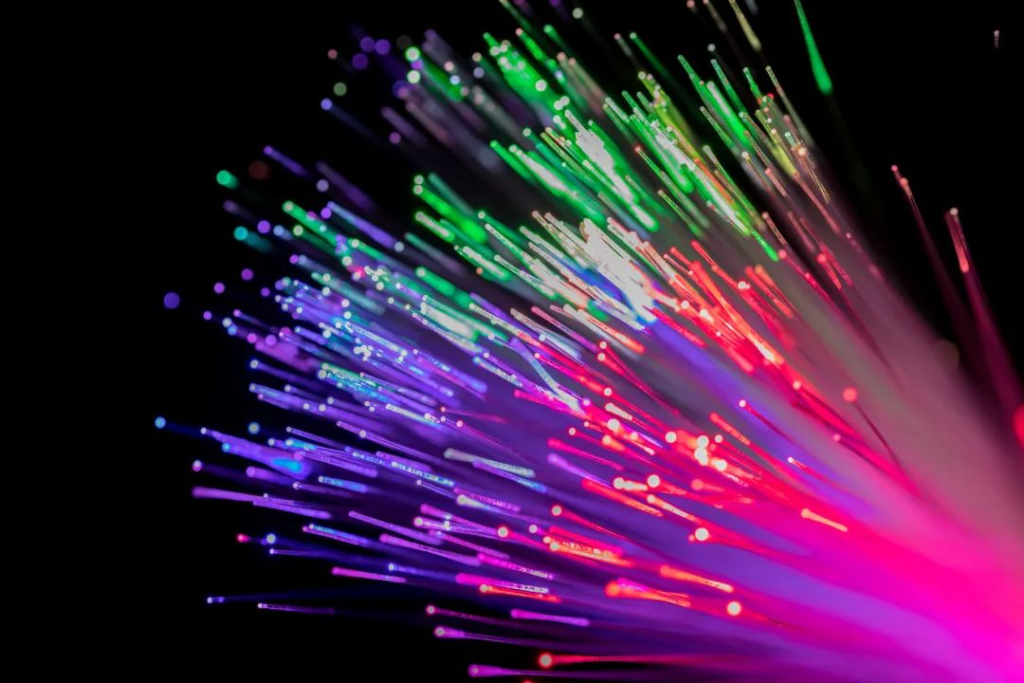
The significance of hollow-core fibers compared to traditional silica-core fibers lies not in cost reduction due to the absence of the solid core, but in the superior propagation of light signals through air rather than glass fibers.
A fundamental formula from high school physics illustrates this advantage:
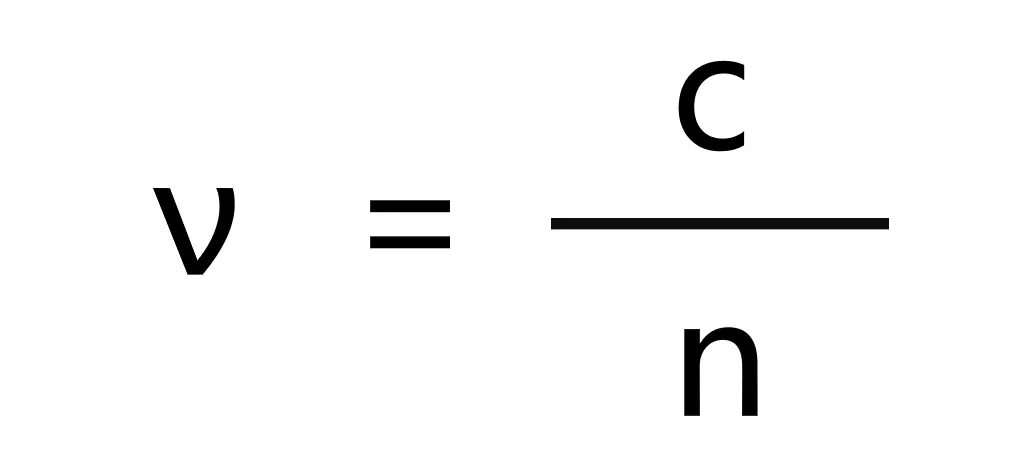
Here, ( v ) represents the speed of light in a medium, ( c ) is the speed of light in a vacuum—commonly known as approximately 300,000 kilometers per second—and ( n ) is the refractive index of the medium. The speed of light varies across different media.
The refractive index of air is approximately 1, while other media have refractive indices greater than 1. For example, water has a refractive index of 1.33, crystal 1.55, and diamond 2.42. Glass varies between 1.5 to 1.9 depending on its composition.
This means that light travels through traditional silica-core fibers at a speed significantly less than ( c ). Experimental data suggests that using hollow-core fibers can increase the speed of light signals by about 47% compared to traditional silica-core fibers.
This increase could substantially reduce the latency in fiber optic communication by about one-third. According to calculations by research institutions, the latency of silica-core fibers is approximately 5 microseconds per kilometer, whereas for hollow-core fibers, it’s about 3.46 microseconds per kilometer. Over a distance of 1000 kilometers, this could reduce latency by 1.54 milliseconds.
Such an improvement in latency is of great importance for industries that rely on high-frequency transactions, such as financial securities trading, as well as for remote healthcare and industrial manufacturing scenarios.
Development and Evolution of Hollow-Core Fiber
Next, let’s examine the technical implementation of hollow-core fiber optics. The principle of optical fibers is essentially about confining light within a cable.
Traditional solid-core optical fibers consist of three parts from the inside out: the core, the cladding, and the coating (sometimes with an additional outer sheath).
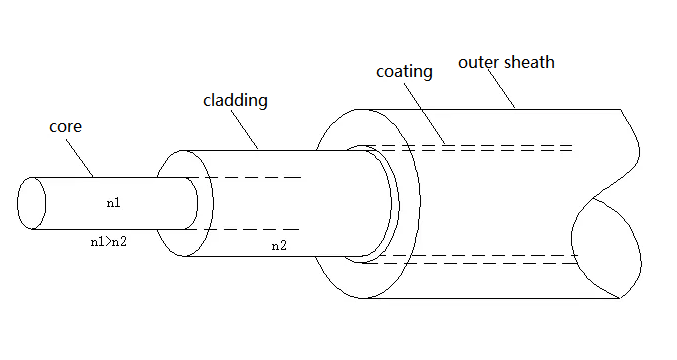
When light enters the optical fiber, the refractive index of the fiber core (n1) is higher than that of the cladding (n2), resulting in total internal reflection. This causes the light to continuously reflect and propagate forward.
In hollow-core fibers, since the refractive index of air is lower than that of the cladding, total internal reflection does not occur.
Therefore, to confine light within hollow-core fibers, a new technological approach is required. As early as the 1960s, when Charles Kao published his seminal paper on optical fibers, the concept of hollow-core fibers was proposed. However, the material technology at that time was not mature enough to realize it.
In 1987, American applied physicists Eli Yablonovitch and Sajeev John first introduced the concept of photonic crystals, breaking the impasse. Photonic crystals, also known as photonic bandgap materials, are artificial microstructures formed by the periodic arrangement of media with different refractive indices.
Simply put, photonic crystals have a “wavelength selection” function, allowing certain wavelengths of light to pass through while blocking others. The iridescent gemstones, butterfly wings, peacock feathers, and beetle shells that exhibit colorful metallic luster in nature all derive their unique optical properties from the periodic microstructures of photonic crystals, which selectively reflect specific wavelengths of light.
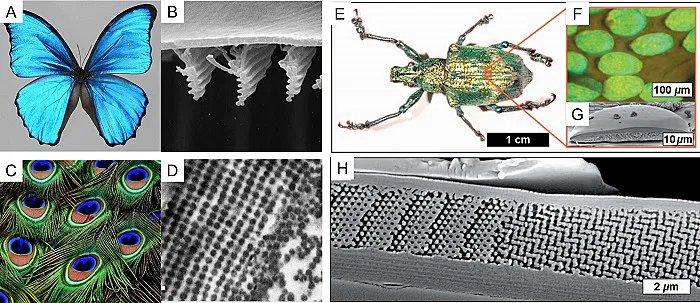
Based on the theory of photonic crystals, in 1991, Philip Russell from the University of Southampton first proposed the concept of photonic crystal fibers (PCFs). In 1996, his colleagues Jonathan Knight and Tim Birks at the Optoelectronics Research Centre of the University of Southampton successfully developed solid-core photonic crystal fiber samples and demonstrated the light transmission characteristics within these fibers.
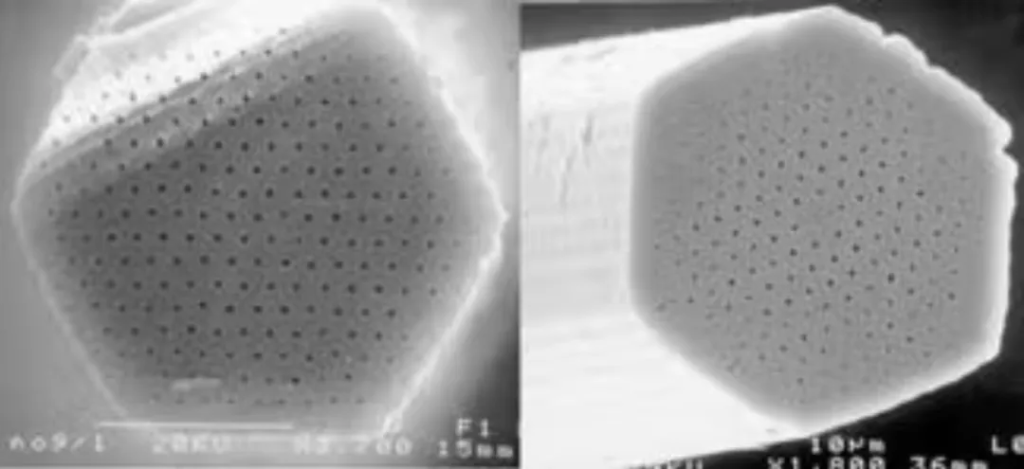
The image above shows the cross-section of an optical fiber at that time. As you can see, there are numerous small holes and no obvious core.
The advent of photonic crystal fibers (PCFs) successfully captured the attention of the optical research community. Many teams began to join the research on PCFs, accelerating related advancements. In 1998, Jonathan Knight and his colleagues announced the discovery of the “photonic bandgap guiding effect in fibers” and produced the world’s first photonic bandgap photonic crystal fiber (PBG-PCF). In 1999, Philip Russell and his team published a paper in Science, proposing the hollow core single-mode photonic bandgap photonic crystal fiber (HC-SM-PBG-PCF). Shortly thereafter, R.F. Cregan and his team successfully developed a sample, which is considered the world’s first hollow-core fiber.
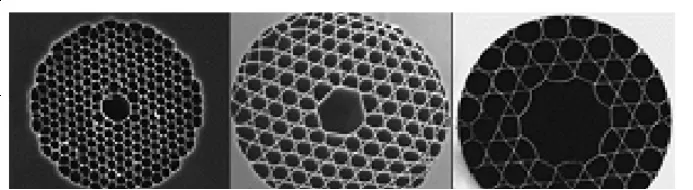
Different structural designs of hollow-core photonic crystal fibers are shown in the image above. The entire PBG-PCF resembles a honeycomb. Therefore, it was also referred to as Holey Fiber (HF) and Micro-Structured Fiber (MSF) at that time. The core of the fiber is hollow and filled with air. The cladding consists of numerous air holes arranged periodically, all with precisely set hole diameters, spacings, and periods. When an optical signal enters the fiber, photons move from the air core into the cladding. The periodically arranged air holes in the cladding form a photonic crystal structure, preventing photons of specific frequencies from passing through the cladding and “bouncing” them back into the core. Thus, photons can only continue to propagate along the air core.
Despite continuous efforts by scientists to improve PBG-PCFs, they have been unable to solve the loss problem. The loss of these fibers has remained at the dB/km level, and their fabrication is challenging. This has hindered the practical application of hollow-core fibers. Consequently, scientists continued to explore new hollow-core fiber structures. Researchers proposed the Kagome-type hollow-core fiber. Later, based on research on Kagome-type hollow-core fibers, they introduced anti-resonant hollow-core fibers, which became a mainstream research direction in the industry.
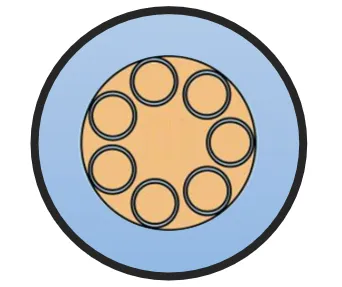
In 2019, Francesco Poletti’s team at the Optoelectronics Research Centre of the University of Southampton invented the renowned Nested Antiresonant Nodeless Fiber (NANF), reducing the loss of hollow-core fibers to 1.3 dB/km. Just a year later, in 2020, Lumenisity, the industrial subsidiary of the University of Southampton, reduced the loss of NANF fibers to 0.28 dB/km, causing a sensation in the industry.
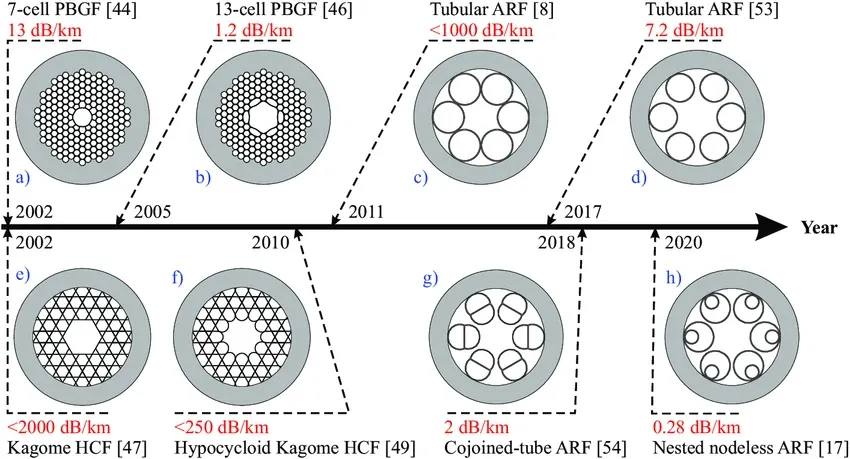
Let’s take a closer look at the structure of NANF fibers:
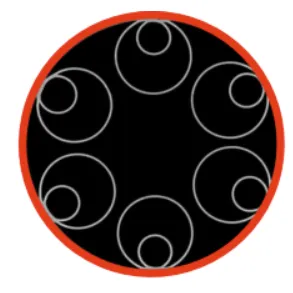
The core of the NANF fiber is filled with air. Surrounding the core are parallel glass tubes, each nested with another glass tube inside. This configuration is known as single nesting. If another tube is nested within, it is referred to as double nesting.
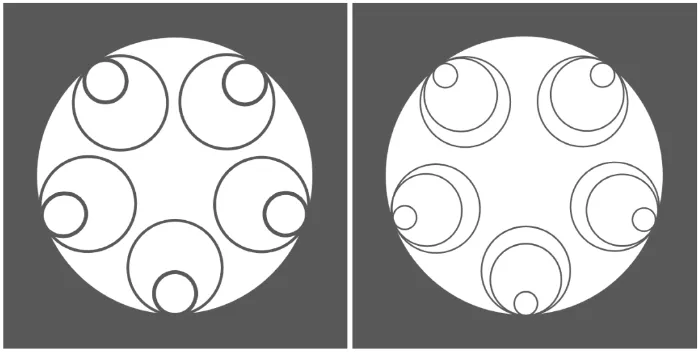
The purpose of nesting is related to “resonance.”
Resonance, also known as interference, occurs when two waves are in sync, resulting in maximum amplitude. Conversely, at certain frequencies, the energy is minimized, known as anti-resonance. The nested glass tubes are designed to form a “resonance cavity.”
The transmission spectrum exhibits multiple peaks. The regions between these peaks are high-reflection zones, also known as anti-resonance windows. Within these windows, light entering from the hollow core will experience high reflection, significantly reducing fiber leakage loss. The sides of the glass tubes do not touch each other, which is referred to as nodeless. If nodes were present, they would cause significant loss.
NANF fibers have overcome the limitations of photonic bandgap photonic crystal fibers (PBG-PCFs) and offer theoretical loss and transmission bandwidth superior to current glass-core fibers, thus attracting significant industry attention.
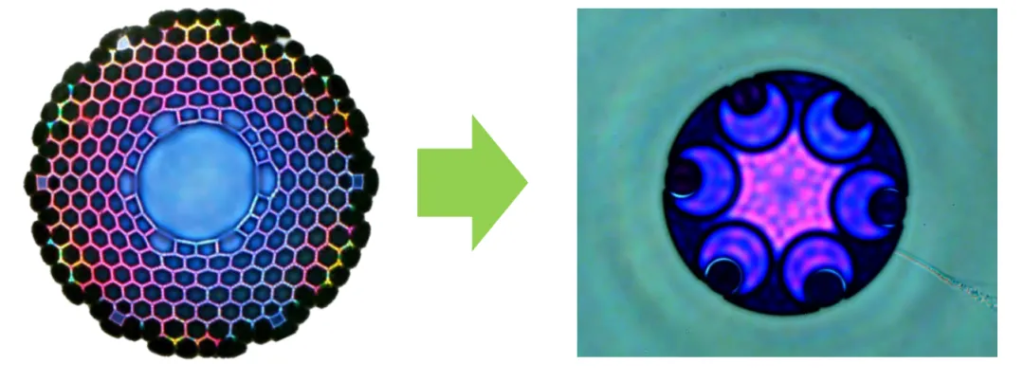
Companies such as BT, Comcast, and euNetworks have adopted Lumenisity’s NANF hollow-core fiber technology in recent years. BT has used NANF for building mobile network backhaul and conducted quantum key distribution tests on NANF. Comcast collaborated with Lumenisity to deploy a 40-kilometer hybrid link of hollow-core and traditional fibers in Philadelphia for compatibility testing. euNetworks deployed a 14-kilometer segment of Lumenisity hollow-core fiber between London and Basildon to connect two data centers critical for financial transactions.
Due to the significant commercial value of hollow-core fibers, Microsoft acquired Lumenisity on December 9, 2022. The transaction price was undisclosed but undoubtedly substantial.
Advantages of Hollow-Core Fibers
Let’s discuss the advantages of hollow-core fibers.
1. Lower Latency
This has been detailed earlier.
2. Lower Loss
Transmission loss is a crucial technical parameter for optical fibers. Lower loss means that the optical signal can travel further within the fiber, making it easier to recognize and demodulate at the receiving end. Optical signals experience less loss when transmitted through air compared to silica glass. As mentioned earlier, current hollow-core fibers can achieve a loss of 0.174 dB/km, comparable to the latest generation of glass-core fibers. According to research institutions, the theoretical minimum loss of hollow-core fibers can be reduced to below 0.1 dB/km, which is lower than that of standard glass-core fibers (0.14 dB/km).
3. Support for Multiple Optical Bands
Hollow-core fibers can easily support various bands such as O, S, E, C, L, and U.
4. Reduced Nonlinear Effects
The nonlinear effects in hollow-core fibers are 3 to 4 orders of magnitude lower than those in conventional glass-core fibers, allowing for significantly higher input optical power and thus extending transmission distances.
5. High-Power Laser Transmission
Traditional glass-core fibers absorb laser energy during high-power laser transmission, leading to heat accumulation at material defects or uneven temperature distribution between the core and cladding, causing fiber damage. In hollow-core fibers, over 99% of the optical power is transmitted through air, minimizing interaction with the material. This results in lower material absorption and a higher laser damage threshold at the same transmission power.
In simple terms, hollow-core fibers are less likely to be damaged by high-power lasers (kilowatt-level).
Besides the advantages listed above, hollow-core fibers also offer low dispersion, low thermal sensitivity, and radiation resistance, which are reasons for the industry’s keen interest in the development of hollow-core fiber technology.
Applications of Hollow-Core Fibers
1. Communication
The low loss and low latency of hollow-core fibers make them ideal for optical communication, especially in latency-sensitive communication scenarios.
2. Sensing
Hollow-core fibers, with their greater flexibility and large aperture, can be used in optical sensing to measure parameters such as temperature, pressure, flow, and chemical composition.
3. Laser Applications
As mentioned earlier, hollow-core fibers can withstand high-power lasers. They can be used to transmit laser beams for industrial manufacturing processes like laser cutting and etching, as well as for imaging and treating diseased tissues deep within the human body. Transmitting lasers is essentially a form of energy transmission, which has significant potential applications.
Conclusion
In summary, hollow-core fibers are highly advantageous and have a broad range of applications. It is essential to increase attention and investment in this technology. Currently, efforts are ongoing to reduce the loss and improve the performance metrics of hollow-core fibers. To accelerate the deployment of this technology, we need to focus on the following points:
1. Standardization of Internal Fiber Structure. Determining the optimal architecture for standardization and mass production.
2. Process Improvement. Reducing manufacturing complexity to achieve large-scale, high-yield production.
3. Engineering Challenges in Deployment. Anticipating and addressing potential engineering issues in real-world deployments, such as how to splice hollow-core fibers if they break.
4. Accelerating Industry Chain Development. Ensuring adequate support in materials and components.
As time progresses, we hope these issues will be resolved, and that hollow-core fibers will soon reach a mature commercial stage, further enhancing our network capabilities.