As we enter a data-driven world, the importance of Ethernet becomes more prominent. Fundamentally, Ethernet is a technology that connects computers to form a local network, through which devices can communicate with other devices. However, over time, Ethernet has evolved into a global data communication system, with speeds ranging from the initial 10Mbit/s to the current 800G, and even 1.6T. This huge progress is not without challenges, but each breakthrough represents a great leap in technology.
What is the QSFP-DD800 Optical Transceiver Module?
QSFP-DD800 stands for Quad Small Form-factor Pluggable Double Density, a high-speed hot-swappable packaging model defined by the QSFP-DD MSA. It is highly compatible with existing fiber optic network equipment, facilitating data center upgrades and expansion.
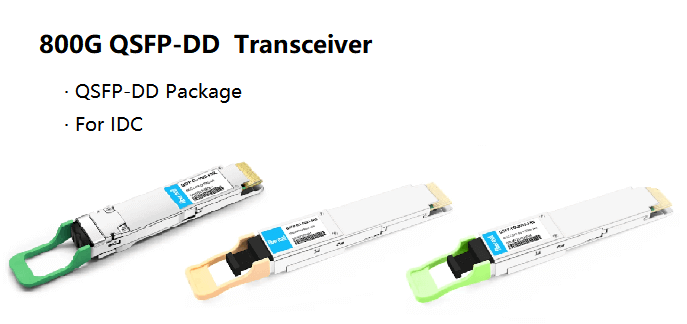
About Transmission Distance
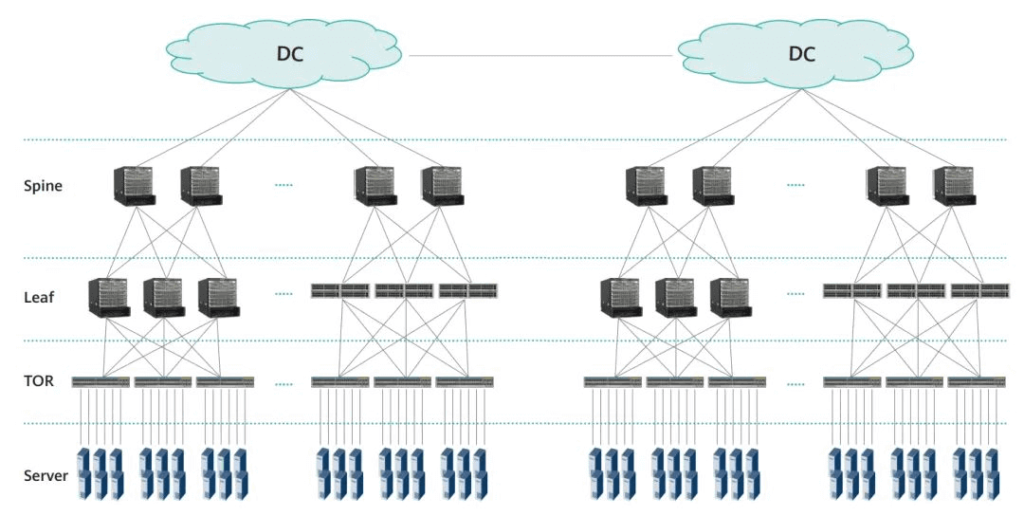
In terms of transmission distance, QSFP-DD800 optical modules support a variety of distance options, which can generally be categorized into VR (50m), SR (100m), DR/FR/LR (500m/2km/10km), etc.
About Optical Interface Types
The optical interface types of QSFP-DD800 optical modules are mainly categorized into MPO, LC, and VSFF (CS/SN/MDC).
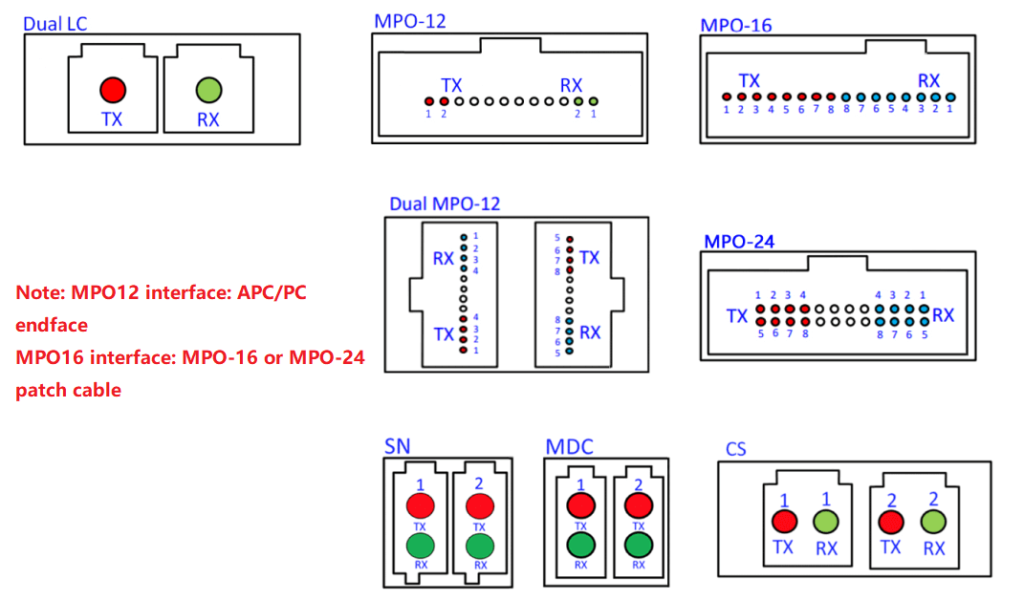
800G Ethernet
800G Ethernet is a high-speed Ethernet technology for data transmission and communication networks, providing a data transfer rate of 800 gigabits per second (800Gbps).
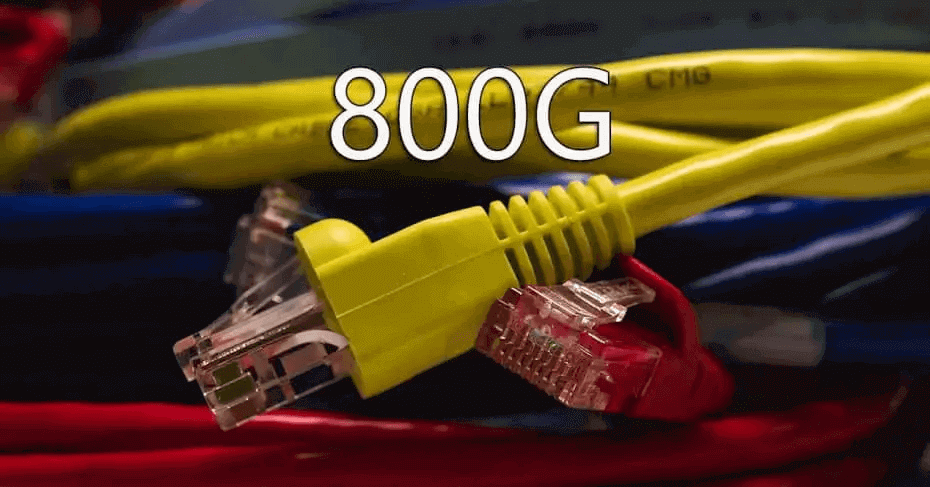
800G Ethernet is twice as fast as the previous generation of 400G Ethernet, offering greater bandwidth for handling large-scale data transfers, high-definition video, cloud computing, Internet of Things, and other high-bandwidth demands. 800G Ethernet uses high-order modulation techniques, typically using PAM4 (pulse amplitude modulation 4) to transmit data, allowing each symbol to carry multiple bits of information, thereby increasing the data transfer rate. 800G Ethernet has important applications in data center networks, where it can improve the interconnection speed between servers within the data center, facilitating large-scale data processing and cloud computing. Achieving 800G Ethernet usually requires advanced network hardware and optical modules that can support high-speed data transmission and typically use low-power design to improve energy efficiency. The standardization of 800G Ethernet is led by IEEE (Institute of Electrical and Electronics Engineers), which helps ensure interoperability between devices from different manufacturers.
The Current State of 800G Ethernet
The current implementation of 800G Ethernet uses 8 channels, each with a transmission rate of 100Gbps. This doubles the PAM4 (four-level modulation) speed from the previous generation of 50Gbps to 100Gbps. The next generation of 800G transceivers under development will increase the speed of each channel to 200Gbps, which brings significant challenges, as it requires simultaneously increasing the high-order modulation and PAM4 data rates.
Challenge One: Switch Silicon SerDes
Faster network switch chips are essential for increasing the channel speed of 800G Ethernet. Network switch chips are used to implement low-latency switching between elements within the data center, which is crucial for supporting high-performance computing and large-scale data transfers. To support the increase in overall switch chip bandwidth, the speed, number, and power of SerDes are also increasing. Currently, SerDes speeds have increased from 10 Gbit/s to 112 Gbit/s, and the number of SerDes channels around the chip has increased from 64 to 512 for a generation of 51.2 Tbps. However, SerDes power consumption has become an important part of the system’s total power consumption. The next generation of switch chips will double the bandwidth again, as 102.4T switches will have 512 200 Gb/s SerDes channels. These silicon switches will support 800G and 1.6T on 224 Gb/s channels.
Solution:
o Faster SerDes: Research and develop faster technologies to meet the growing data transfer demand. This includes increasing the speed, reducing the power consumption, and improving the signal integrity of SerDes.
o Power optimization: Adopt a power-optimized design approach to reduce the power consumption of SerDes. This includes using advanced CMOS processes and low-power circuit design.
Challenge Two: Pulse Amplitude Modulation
High-order modulation increases the number of bits per symbol or unit interval (UI), providing a trade-off between channel bandwidth and signal amplitude. Standards often explore higher-order modulation schemes to increase data rates. PAM4 modulation is backward compatible with previous generations of products, and compared with higher modulation schemes, it provides a better signal-to-noise ratio (SNR), thereby reducing the forward error correction (FEC) overhead that causes latency. However, due to analog bandwidth limitations and advanced equalization achieved through innovative DSP schemes, PAM4 requires a better analog front-end (AFE).
Solution:
o Better analog front-end (AFE): Research and develop higher-performance analog front-ends to support higher-order modulation schemes. This may include more accurate clock recovery, lower jitter, and better signal processing capabilities.
o Advanced equalization techniques: Use innovative digital signal processing (DSP) and equalization techniques to overcome distortion and noise in the channel. This helps improve the reliability of PAM4 signals.
o Explore higher modulation solutions: Although PAM4 is widely used in current 800G Ethernet, future standards may adopt higher-order modulation schemes, such as PAM6 or PAM8. This will increase the transmission rate per symbol but also bring higher complexity.
How to Reduce the Bit Error Rate of 800G Ethernet?
In high-speed data transmission, the signal is affected by various interference and attenuation factors when passing through the channel. These include signal attenuation, noise, crosstalk, and other signal distortion factors. These factors cause bit errors in the signal, which are called bit errors. The presence of bit errors during data transmission may cause serious data corruption, reducing the availability and integrity of data. In previous high-speed data standards, such as 100G Ethernet, conventional fine-tuning equalizers and signal processing techniques were sufficient to reduce the bit error rate. However, in the higher-speed 800G Ethernet, more complex methods are needed to cope with the higher bit error rate challenge.
Forward Error Correction (FEC) Algorithm
Forward error correction (FEC) is a widely used technique to reduce the bit error rate. It involves adding redundant information to data transmission to help the receiver detect and correct errors in transmission. FEC algorithms add redundant bits to data frames, allowing the receiver to reconstruct lost or damaged data bits. This helps improve the reliability of data transmission, especially in high-speed networks.
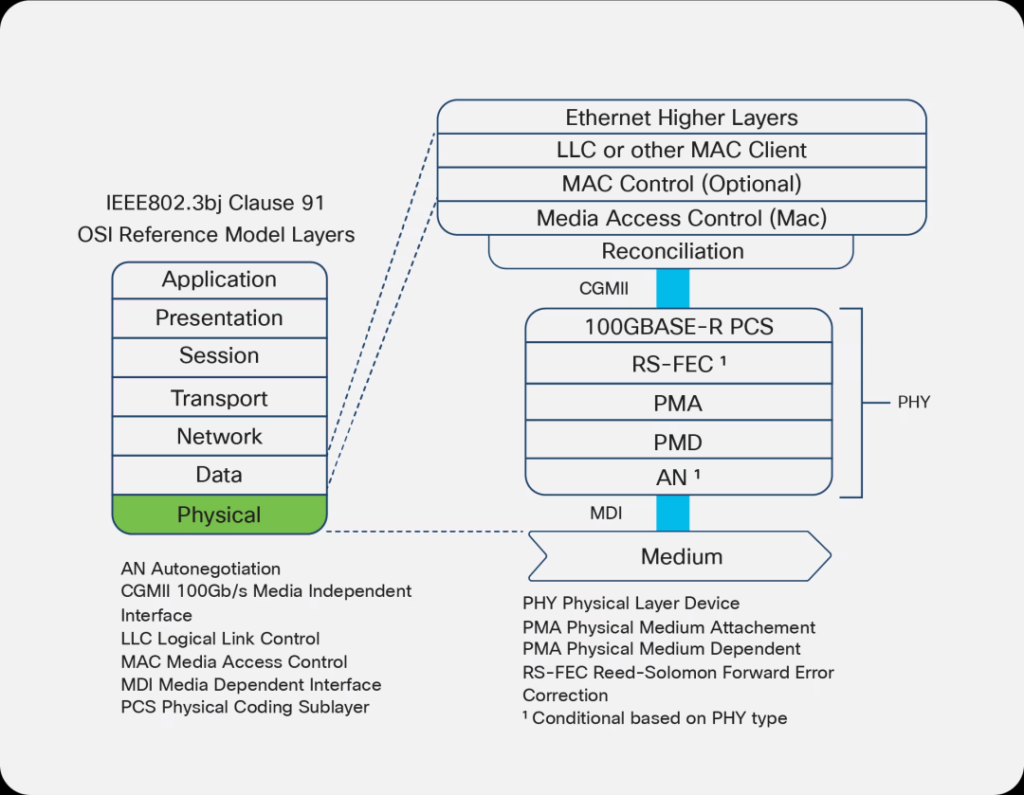
The Importance of FEC
FEC becomes especially important in high-speed networks such as 800G Ethernet. Due to the higher data rates, the bit error rate in transmission is usually higher. Therefore, more powerful FEC algorithms are needed to minimize the bit error rate and to ensure the reliability of high-speed networks.
The Trade-Offs and Advantages of FEC
Each FEC architecture involves trade-offs and advantages in terms of coding gain, overhead, latency, and power efficiency. Here are some common FEC architectures and their characteristics:
Reed-Solomon Coding
Reed-Solomon coding is a widely used FEC technique in data storage and communication. It has good error correction performance and can recover data frames from random errors. However, it requires relatively large redundancy, which may introduce large overhead in high-speed networks.
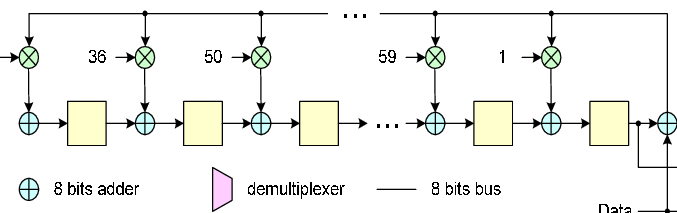
LDPC (low-density parity-check) coding
LDPC coding is an efficient FEC technique that is widely used in high-speed networks. It has low coding overhead and performs well in reducing the bit error rate. LDPC coding also has low latency and power consumption.
BCH coding
BCH coding is a suitable FEC technique for high-speed communication, which achieves a balance between error correction performance and coding overhead. It is commonly used in optical fiber communication and high-speed data storage.
Complex FEC Algorithms
In 224 Gb/s systems, more complex FEC algorithms are needed to cope with the higher bit error rate challenge. These algorithms may include using more redundant data and more sophisticated error correction mechanisms to ensure the reliability of data transmission.
How to Improve the Energy Efficiency of 800G Ethernet?
The power consumption of each generation of optical modules is increasing, especially in high-speed networks such as 800G and 1.6T Ethernet. Although optical module design has become more efficient, reducing the power consumption per bit, due to the large data centers that usually have tens of thousands of optical modules, the overall power consumption of the modules is still a serious problem.
Energy Efficiency Challenge
Improving the energy efficiency of 800G Ethernet is an important challenge, especially in large-scale data centers. The energy consumption of data centers has important impacts on cost, environment, and sustainability. Therefore, reducing the power consumption of 800G Ethernet devices is crucial.
Co-Packaged Optics
One way to solve the power consumption challenge of optical modules is to use co-packaged optics. This technology reduces the power consumption of each module by integrating the optoelectronic conversion function within the optical module package. Co-packaged optics can provide various advantages, such as higher energy efficiency and smaller package sizes.
Advantages of Co-Packaging Technology
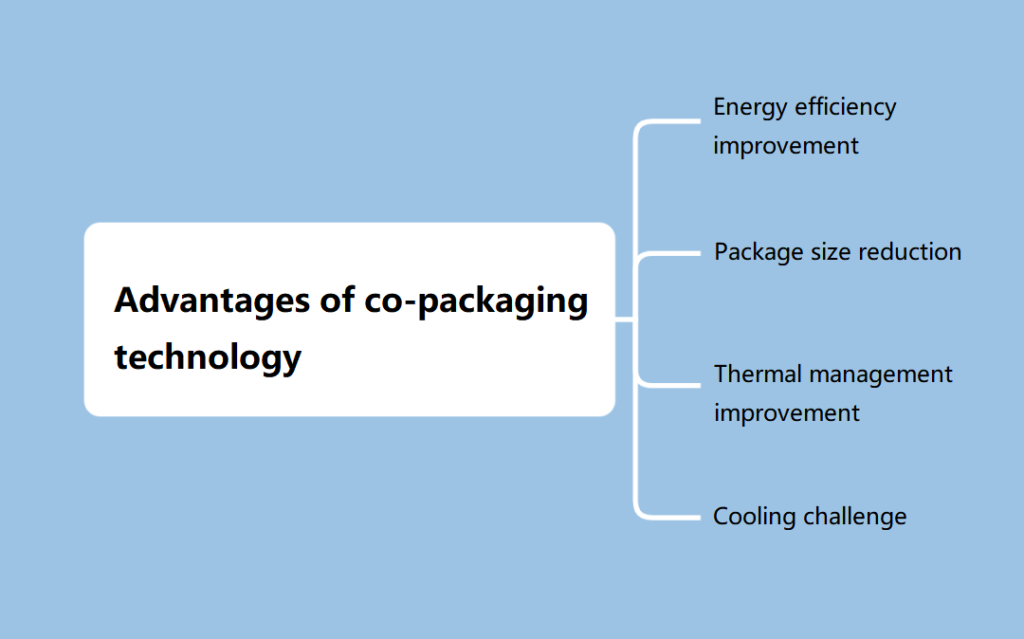
Energy efficiency improvement
Co-packaged optics can improve energy efficiency by integrating the optoelectronic conversion function into the optical module. This integration reduces the energy loss in the process of optical signal conversion and transmission. Therefore, the power consumption per bit is reduced, while providing higher energy efficiency.
Package size reduction
Co-packaging technology can also reduce the package size of optical modules. This is especially important for large data centers, as they need more devices to be placed in a limited space. Smaller package sizes can improve the scalability and layout flexibility of data centers.
Thermal management improvement
Due to the lower power consumption, co-packaged optics generate less heat. This helps improve the thermal management of data centers, reducing the cooling demand and lowering the operating costs.
Cooling Challenge
However, co-packaged optics also bring new challenges, one of which is cooling. The heat generated by the integrated optoelectronic converters inside the package needs to be effectively dissipated to prevent overheating and performance degradation. Therefore, designing efficient cooling solutions is essential for the success of co-packaging technology.
1.6T Ethernet
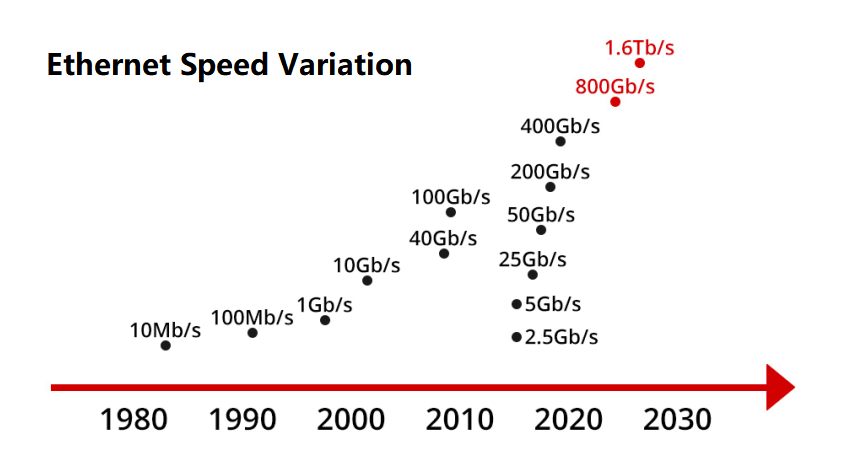
1.6T Ethernet is a high-speed Ethernet technology for data transmission and communication networks, providing a data transfer rate of 1.6 terabits per second (1.6Tbps). It represents the latest development in the network field and is an upgrade from 800G Ethernet. 1.6T Ethernet is twice as fast as 800G Ethernet, offering greater bandwidth. It is suitable for handling large-scale data transfers, high-definition video, cloud computing, high-performance computing, and other demands for extremely high bandwidth. 1.6T Ethernet uses higher-order modulation techniques, typically using PAM4 (pulse amplitude modulation 4) or higher-order modulation methods to transmit data, to achieve higher data transfer rates.
1.6T Ethernet has important applications in data center networks and network backbone. It can meet the high-speed interconnection needs between servers within large data centers, and also support a higher-speed network backbone to connect different data centers and network nodes.
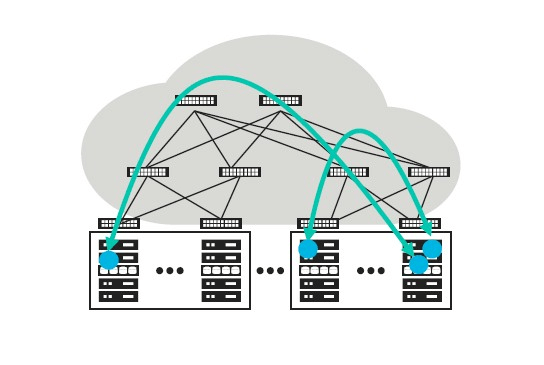
Timeline of 800G Ethernet and 1.6T Network
The development of 800G Ethernet is based on the previous generation of 400G Ethernet. In the past few years, standard organizations such as IEEE (Institute of Electrical and Electronics Engineers) and OIF (Optical Internetworking Forum) have established standards for 400G networks, laying the foundation for the development of 800G. 1.6T network is a further development of 800G Ethernet, representing a higher-speed network technology. Although the development of the 1.6T network is still in its early stages, it has attracted widespread attention.
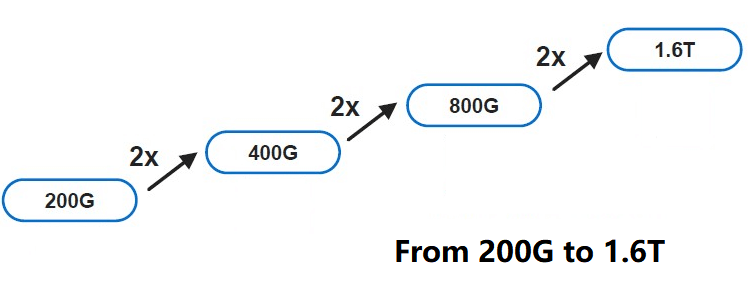
2022: The first 51.2T switch chip is released
In 2022, the network industry achieved an important milestone, which was the release of the first 51.2T switch chip. These switch chips support 64 ports of 800Gb/s, marking the development of 800G Ethernet entering the practical hardware stage. At the same time, this period also witnessed the start of the verification work for the first batch of 800G optical modules.
2023: Standard release and development verification
In 2023, standard organizations made significant progress. First, IEEE released the first version of the IEEE 802.3df standard, which defines the physical layer specifications for 800G Ethernet. Meanwhile, OIF also released the 224 Gb/s standard, which provides guidance for building 800G and 1.6T systems with 112 Gb/s and 224 Gb/s channels.
The next two years: Final determination of physical layer standards
In the next two years, it is expected that standard organizations will continue to work hard to finalize the physical layer standards for 800G Ethernet. This will involve further refinement and testing of the specifications to ensure the interoperability and performance of network devices. Although the timetable for the 1.6T network is not clear yet, it is regarded as part of the future network development. With the continuous development of the digital era, the demand for higher speed and larger capacity will continue to grow, and the 1.6T network is expected to meet these needs.
Multiple Application Scenarios of 800G and 1.6T Ethernet
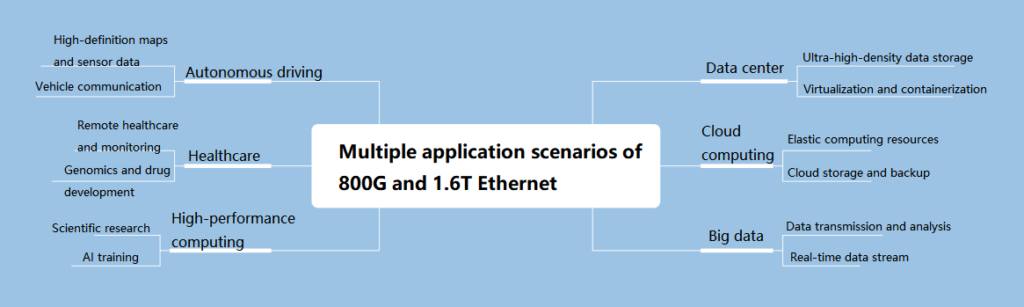
Data center
Ultra-high-density data storage
Data centers need a lot of storage capacity and fast data transmission to meet the growing data demand. 800G and 1.6T Ethernet can be used to connect storage servers and achieve ultra-high-density data storage. For example, a large social media company can use these high-speed Ethernet technologies to support the massive amount of photos and videos uploaded by users.
Virtualization and containerization
Virtualization and containerization technologies require fast data transmission to share resources among virtual machines or containers. 800G and 1.6T Ethernet can be used to provide high-bandwidth virtual machine migration and container communication. For example, a cloud service provider can use these technologies to support the virtualization workloads of customers.
Cloud computing
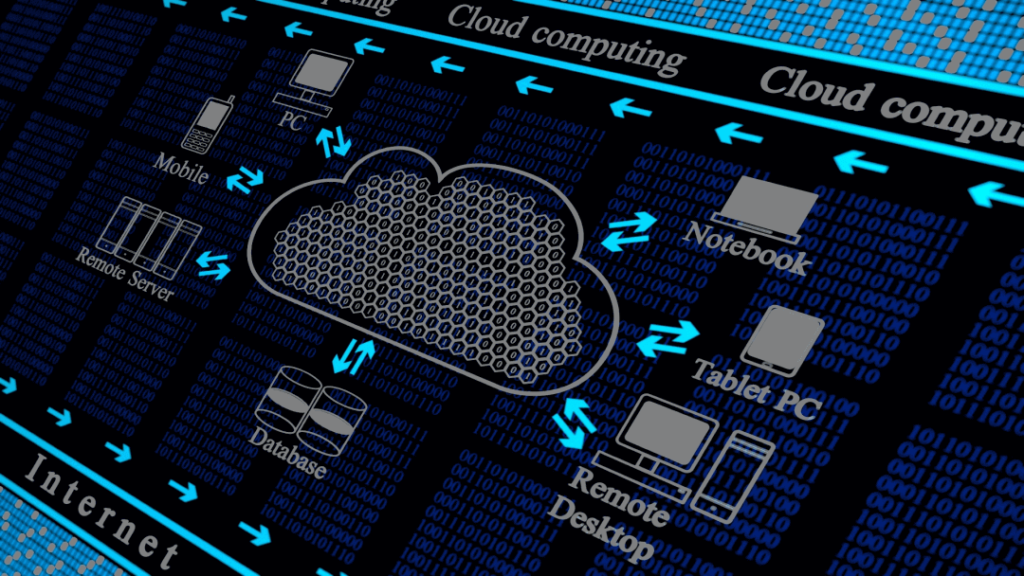
Elastic computing resources
Cloud computing provides the ability of elastic computing resources, but this requires high-speed network connections. 800G and 1.6T Ethernet can be used to provide fast data transmission between cloud computing users. For example, a scientific research institution can use these high-speed network connections to run complex simulations and data analysis tasks in the cloud.
Cloud storage and backup
Cloud storage and backup services need large capacity and high-speed transmission to ensure the security and availability of data. These high-speed Ethernet technologies can be used to connect cloud storage devices and data backup servers. For example, an enterprise can use them to back up important business data.
Big data
Data transmission and analysis
Big data analysis requires a lot of data transmission and processing capabilities. 800G and 1.6T Ethernet can be used to transfer large-scale data sets from data sources to analysis platforms and accelerate the data processing process. For example, a healthcare organization can use these high-speed networks to analyze many medical records of patients to improve diagnosis and treatment.
Real-time data stream
Real-time data stream processing requires data to be transmitted in the network with extremely low latency. These high-speed Ethernet technologies can be used to support real-time data stream applications, such as financial transaction monitoring and smart city monitoring. For example, a financial institution can use them to monitor and analyze a large amount of transaction data to detect potential fraud activities.
High-performance computing
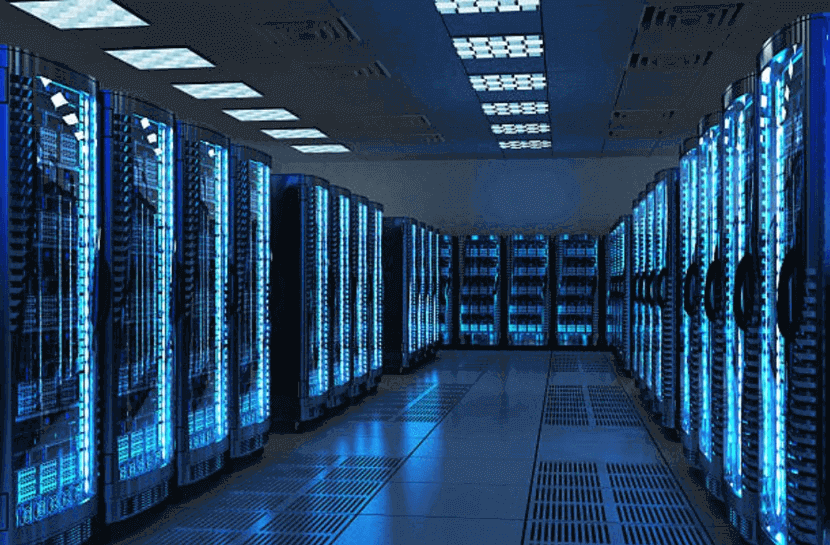
Scientific research
High-performance computing is used to solve complex problems in science and engineering fields. 800G and 1.6T Ethernet can be used to connect supercomputers and data centers, to support scientists in simulation and model computation. For example, an aerospace company can use these high-speed networks to simulate the performance and safety of aircraft.
Artificial intelligence training
Artificial intelligence training requires a lot of data transmission and computation capabilities. These high-speed Ethernet technologies can be used to connect GPU clusters and data storage, to support the training of deep learning models.
Healthcare
Remote healthcare and monitoring
In the future, remote healthcare and monitoring will be a major trend. 800G and 1.6T Ethernet technologies will support high-quality remote healthcare services, including remote surgery and patient monitoring.
Genomics and drug development
The healthcare sector needs massive data processing capabilities for genomics research and drug development. High-speed Ethernet will be used to transmit large amounts of gene and drug data, accelerating medical research.
Autonomous driving
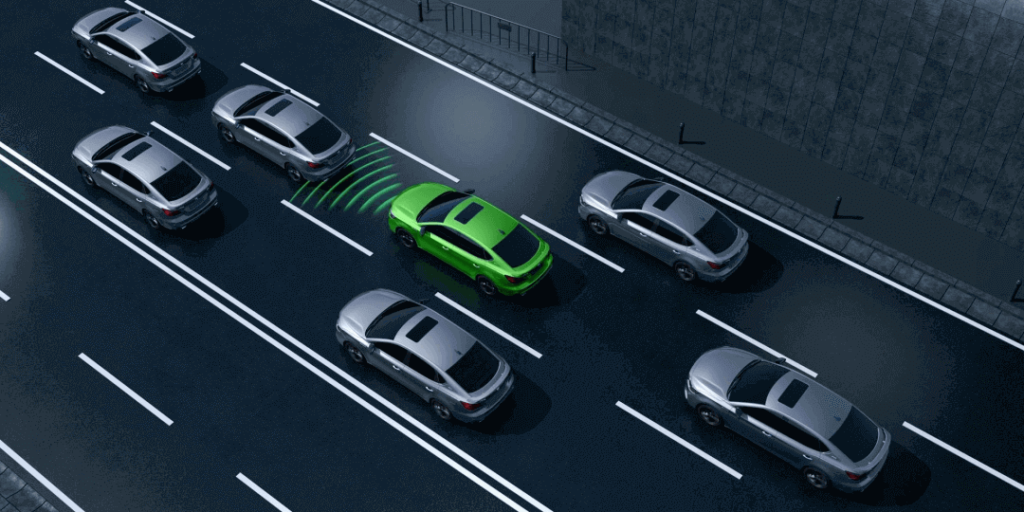
High-definition maps and sensor data
Autonomous vehicles need high-resolution maps and sensor data to achieve accurate positioning and environmental perception. 800G and 1.6T Ethernet technologies will be used to transmit these large-scale data, improving the safety and reliability of autonomous driving.
Vehicle communication
Communication between vehicles and between vehicles and infrastructure will be critical for autonomous driving. High-speed Ethernet will support real-time communication between vehicles, helping to avoid collisions and improve traffic efficiency.
Conclusion
The emergence of 800G and 1.6T Ethernet is a significant technological innovation. They will enable us to handle larger data payloads and meet higher performance requirements. 400G is being deployed on a large scale, but there is still a long way to go before reaching the data rate of 800G, and the optimal path for 1.6T is still uncertain. In just a few years, there will undoubtedly be a need for higher capacity, faster speed, and significant efficiency improvements. To prepare for the expansion of these new technologies, it is necessary to start designing and planning from today.
Related Products:
-
NVIDIA MMS4X00-NM-FLT Compatible 800G Twin-port OSFP 2x400G Flat Top PAM4 1310nm 500m DOM Dual MTP/MPO-12 SMF Optical Transceiver Module $900.00
-
NVIDIA MMA4Z00-NS-FLT Compatible 800Gb/s Twin-port OSFP 2x400G SR8 PAM4 850nm 100m DOM Dual MPO-12 MMF Optical Transceiver Module $650.00
-
NVIDIA MMA4Z00-NS Compatible 800Gb/s Twin-port OSFP 2x400G SR8 PAM4 850nm 100m DOM Dual MPO-12 MMF Optical Transceiver Module $650.00
-
NVIDIA MMS4X00-NM Compatible 800Gb/s Twin-port OSFP 2x400G PAM4 1310nm 500m DOM Dual MTP/MPO-12 SMF Optical Transceiver Module $900.00
-
OSFP-XD-1.6T-4FR2 1.6T OSFP-XD 4xFR2 PAM4 1291/1311nm 2km SN SMF Optical Transceiver Module $15000.00
-
OSFP-XD-1.6T-DR8 1.6T OSFP-XD DR8 PAM4 1311nm 2km MPO-16 SMF Optical Transceiver Module $12000.00
-
OSFP-800G-FR4 800G OSFP FR4 (200G per line) PAM4 CWDM Duplex LC 2km SMF Optical Transceiver Module $3500.00
-
OSFP-800G-2FR2L 800G OSFP 2FR2 (200G per line) PAM4 1291/1311nm 2km DOM Duplex LC SMF Optical Transceiver Module $3000.00
-
OSFP-800G-DR4 800G OSFP DR4 (200G per line) PAM4 1311nm MPO-12 500m SMF DDM Optical Transceiver Module $3000.00
-
QSFP-DD-800G-2FR2 800G QSFP-DD800 2FR2 (200G per line) 2km 1291/1311nm Dual CS SMF Transceivers $3000.00
-
QSFP-DD-800G-SR8 800G SR8 QSFP-DD 850nm 100m OM4 MMF MPO-16 Optical Transceiver Module $850.00
-
QSFP-DD-800G-2FR4 800G QSFP-DD 2FR4 PAM4 1310nm 2km DOM Dual CS SMF Optical Transceiver Module $1900.00
-
QSFP-DD-800G-DR8 800G-DR8 QSFP-DD PAM4 1310nm 500m DOM MTP/MPO-16 SMF Optical Transceiver Module $1300.00
-
QSFP-DD-800G-FR8 QSFP-DD 8x100G FR PAM4 1310nm 2km DOM MPO-16 SMF Optical Transceiver Module $1500.00
-
QSFP-DD-800G-LR8 QSFP-DD 8x100G LR PAM4 1310nm 10km MPO-16 SMF FEC Optical Transceiver Module $1600.00
-
QSFP-DD-800G-FR8L QSFP-DD 800G FR8 PAM4 CWDM8 2km DOM Duplex LC SMF Optical Transceiver Module $3000.00
-
OSFP-800G-DR8 OSFP 8x100G DR PAM4 1310nm MPO-16 500m SMF DDM Optical Transceiver Module $900.00
-
OSFP-800G-FR8 OSFP 8x100G FR PAM4 1310nm MPO-16 2km SMF Optical Transceiver Module $1200.00
-
OSFP-800G-2LR4 OSFP 2x400G LR4 PAM4 CWDM4 Dual CS 10km SMF Optical Transceiver Module $2000.00
-
OSFP-800G-SR8D-FLT OSFP 8x100G SR8 Flat Top PAM4 850nm 100m DOM Dual MPO-12 MMF Optical Transceiver Module $650.00