The latest trend in backbone network communications is the adoption of 400Gbps technology. This significant upgrade has been rolling out since last year, with a full-scale launch of commercial 400G services in 2023 and a planned expansion into mass-market applications in 2024. A notable milestone was reached in March 2024 when China Mobile launched the world’s first 400Gbps all-optical transcontinental (Beijing to Inner Mongolia) backbone line.
The transition to 400Gbps backbone networks is driven by several factors. The increasing demand for high-speed internet due to the rise in digital lifestyles, such as high-definition video streaming, remote meetings, live streaming, and online gaming, continues to grow. Additionally, the push for digital transformation across industries has led to a surge in data traffic from digital systems, further straining backbone networks.
Another critical factor is the AI boom. Following the emergence of large AI models, there has been a wave of AI development. To meet the demands of AI businesses, numerous data centers are being constructed. These models have evolved from having billions of parameters to tens of billions, requiring GPU computing clusters to scale up from thousands to tens of thousands of teraflops.
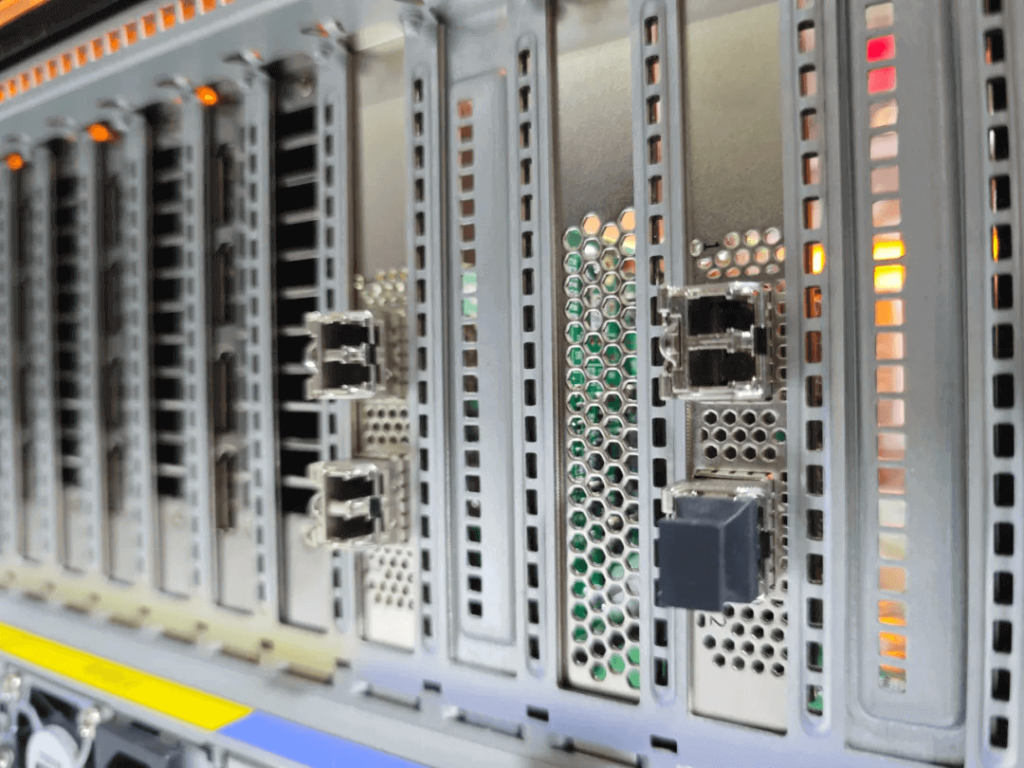
Previously, GPU computing clusters were part of the data center’s internal network (DCN). However, as these clusters grow in size, there is a shift towards deploying distributed data centers for model training. This change places higher demands on data center interconnect networks (DCI), necessitating backbone optical networks that can meet these technical performance requirements.
China’s strategic approach to computational power remains focused on “national coordination and comprehensive planning.” Since February 2022, the East-West Computing Project has been initiated to build a unified national computational power system. In essence, this involves constructing numerous data centers (akin to power plants) and robust backbone transmission networks (similar to power grids) to distribute computational power and meet the diverse needs of various industries.
The implementation of 400Gbps in backbone network communications is a result of advancements in several key areas. The backbone network, which serves as the foundation for our digital society, must possess ultra-high bandwidth (400Gbps and potentially 800Gbps or 1.6Tbps), ultra-low latency (multi-level latency rings), ultra-large-scale meshing (serving distributed computing and AI clusters), ultra-high reliability, ultra-high availability, ultra-high security, ultra-flexible deployment, and intelligent operation and management.
To achieve the current speeds of 400Gbps, the following aspects have been crucial:
Firstly, the baud rate. The transmission rate is measured in bits per second (bps) and represents the number of bits transmitted in a given time period.
Bit rate = Baud rate * Number of binary bits per symbol
The baud rate is determined by the number of symbols transmitted per unit time. A higher symbol rate means more symbols are transmitted per second, resulting in a larger information quantity and higher speed.
The baud rate is limited by the capabilities of optical components. As semiconductor fabrication technology advances from 16nm to 7nm and 5nm, the symbol rate has increased from over 30 Gbaud to 64 Gbaud, 90 Gbaud, and 128 Gbaud. The current commercial use of 400Gbps is made possible by achieving a symbol rate of 128 Gbaud.
Secondly, the modulation scheme.
The modulation schemes used for 400G technology include 16QAM, 16QAM-PCS (PCS stands for Probabilistic Constellation Shaping, which will be discussed in detail later), and QPSK. These schemes are suitable for different applications.
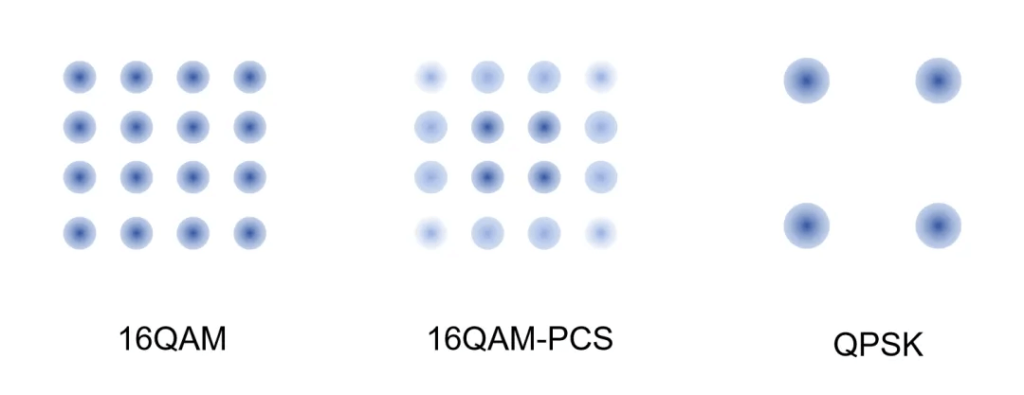
Optical communication and wireless communication differ in their approach to higher-order modulation. Lower-order modulation requires less stringent requirements for the transmission *6-+initially focused on 16QAM and QPSK. With the introduction of 16QAM-PCS, competition increased.
Initially, there was no mention of “East-West Computing” or that operators believed 400G would not require long-distance transmission. Thus, more mature and cost-effective low-symbol-rate components were used in conjunction with high-order modulation schemes like 16QAM, which became the industry standard.
However, as the distance requirements for transmission increased from over 1000km to several thousand km, and with the rapid maturation of 128 Gbaud symbol rate components (which also led to the swift emergence of 800Gbps in DCN scenarios), conditions were created for QPSK to stand out.
QPSK has a higher tolerance for non-linearity compared to 16QAM-PCS, allowing for an appropriate increase in launch power into the fiber. Additionally, QPSK’s backward OSNR (Optical Signal-to-Noise Ratio) limit is better optimized than that of 16QAM-PCS. Furthermore, setting QPSK’s channel spacing at 150GHz ensures that there is almost no filtering cost during transmission.
These advantages have made QPSK increasingly popular as the industry’s preferred choice for backbone networks and DCI.
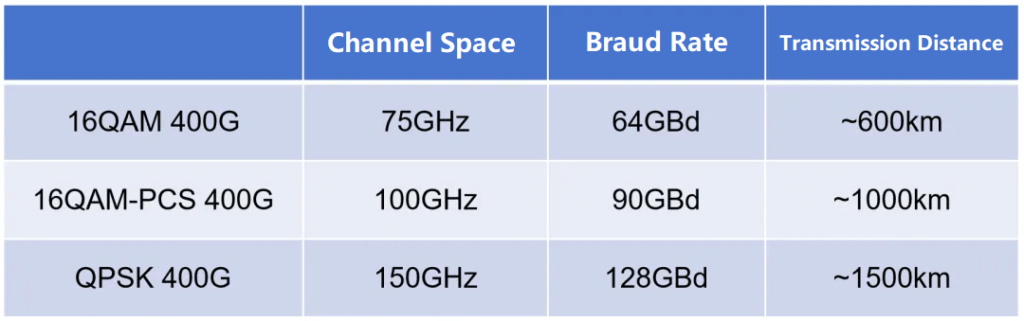
The focus of consideration for the first two scenarios has been on urban or provincial applications. The third scenario involves expanding the bandwidth.
The bandwidth and modulation primarily affect the speed of a single channel. A single fiber can carry multiple waves, provided the frequency spectrum is sufficiently broad. The formula for calculating the bandwidth of a single fiber is:
Single Fiber Bandwidth=Single Channel Bandwidth×Number of Channels
For example, QPSK 400G has a channel spacing of 150GHz. Traditional C-band and extended C-band are insufficient to meet the bandwidth requirements.
Therefore, there is a gradual shift towards using C6T+L6T, which provides a total bandwidth of 12THz. With 80 channels, each operating at 400G, this results in a single fiber capacity of 32Tbps. If some distance is sacrificed for use in provincial areas, employing QPSK or 16QAM-PCS can further increase capacity to 48Tbps.
The main challenge with bandwidth expansion is whether the equipment can support it and whether the costs are controllable. The equipment in question includes ITLA, CDM, ICR, EDFA, and WSS, which involve aspects of light reception and transmission, as well as optical switching and amplification.
Bandwidth expansion also raises the issue of integration.
Currently, bandwidth expansion is more akin to the simple binding of two systems (C and L). These systems operate independently and are transmitted through combined waves. Upon reaching the destination, they are demultiplexed and processed separately.
This approach results in larger volumes, higher power consumption, and more complex designs. Therefore, the industry must research how to integrate components to allow a single system to support different bandwidth expansions, achieving true system integration.
In addition to modulators and optical devices, fiber optics also require attention.
The mainstream fiber currently in use is G.652D fiber. 400G QPSK on G.652D fiber can be amplified using EDFA to achieve transmission distances of up to 1500km.
After years of verification by the industry, G.654E fiber has been established as its successor. If G.654E fiber with better performance is used under the same conditions, the transmission distance for 400G QPSK can increase by more than 30%.
G.654E fiber has already achieved mass production capability and will be deployed on long-haul backbone lines. Some low-loss fibers from the G.654 series have become the preferred choice for transoceanic cable systems over long distances.
Beyond traditional fibers, the industry believes that multi-core fibers and hollow-core fibers have broad application prospects.
Multi-core fibers are a form of space-division multiplexing within a single fiber by inserting multiple cores; they adopt a low-modulation format to significantly increase the capacity of optical fibers.
Hollow-core fibers take it a step further by making the fiber hollow and replacing the glass core with air.
Hollow-core fibers have been proven to offer greater capacity, lower latency, smaller transmission loss, and extremely low nonlinearity; they are unanimously recognized by the industry as having significant potential in optical communication.
800G or 1.6T
The next step for 400G after its commercial rollout is to focus on technologies beyond 400G (beyond 400G) within technical standard systems.
The industry is currently debating whether to pursue 800G or 1.6T.
To achieve higher speeds, further development must occur in “modulation method + bit rate.” For instance, 130GBd or higher bit rates are inevitable directions. Higher bit rates necessitate corresponding equipment advancements to form a mature industry chain.
Exceeding 400G means QPSK modulation is no longer sufficient; 16QAM modulation is currently widely accepted in the industry.
Bandwidth also needs further expansion. Building upon C+L’s foundation, consideration should be given to extending into S-band, U-band, E-band, etc., which would result in a combined bandwidth of 12T+5T or 17THz.
Considering multiple factors, it is conceivable that a single fiber could achieve single-direction transmission speeds exceeding 100Tbps within reach.
Within data centers’ internal networks, 800G (based on bit rates exceeding 100GBd for single-channel operation) has already been put into commercial use. Single-channel speeds of 200G, 400G, and 800G are only a matter of time; progress in this area has been faster internationally.
As capacity continues to grow exponentially, so do the technological challenges. The development of optical communication relies heavily on components such as devices and chips; process technology; materials.
To meet requirements related to power consumption; safety; maintenance; etc., reliance also extends to manufacturing processes; architecture; packaging; artificial intelligence; digital twins.
Much work remains for upstream and downstream sectors within the industry chain.
The future path remains long.
Summary
Optical communication is the digital artery of the whole society. Over the years, people have questioned many technologies (including 5G), but no one will question optical communication because it is an immediate need for the development of society.
The trend of increasing human data traffic is unchanged for decades to come. The rapid rise of artificial intelligence technology will amplify this trend even further.
The current development of optical communication is unable to meet the demand. This means that companies will have a greater incentive to invest resources in research and development in order to make profits.
Related Products:
-
NVIDIA MMS1Z00-NS400 Compatible 400G NDR QSFP112 DR4 PAM4 1310nm 500m MPO-12 with FEC Optical Transceiver Module $800.00
-
NVIDIA MMS4X00-NS400 Compatible 400G OSFP DR4 Flat Top PAM4 1310nm MTP/MPO-12 500m SMF FEC Optical Transceiver Module $800.00
-
NVIDIA MMA1Z00-NS400 Compatible 400G QSFP112 SR4 PAM4 850nm 100m MTP/MPO-12 OM3 FEC Optical Transceiver Module $650.00
-
NVIDIA MMA4Z00-NS400 Compatible 400G OSFP SR4 Flat Top PAM4 850nm 30m on OM3/50m on OM4 MTP/MPO-12 Multimode FEC Optical Transceiver Module $650.00
-
QSFP-DD-400G-SR8 400G QSFP-DD SR8 PAM4 850nm 100m MTP/MPO OM3 FEC Optical Transceiver Module $180.00
-
QSFP-DD-400G-DR4 400G QSFP-DD DR4 PAM4 1310nm 500m MTP/MPO SMF FEC Optical Transceiver Module $450.00
-
QSFP-DD-400G-ER4 400G QSFP-DD ER4 PAM4 LWDM4 40km LC SMF without FEC Optical Transceiver Module $4500.00
-
QSFP-DD-400G-SR4 QSFP-DD 400G SR4 PAM4 850nm 100m MTP/MPO-12 OM4 FEC Optical Transceiver Module $600.00
-
LQSFP112-400G-DR4 400G LPO QSFP112 DR4 PAM4 1310nm 500m MTP/MPO-12 with KP4 FEC Optical Transceiver Module $1215.00
-
LQSFP112-400G-SR4 400G LPO QSFP112 SR4 PAM4 850nm 50m MTP/MPO-12 OM3 FEC Optical Transceiver Module $890.00
-
QSFP112-400G-DR4 400G QSFP112 DR4 PAM4 1310nm 500m MTP/MPO-12 with KP4 FEC Optical Transceiver Module $1350.00
-
QSFP112-400G-SR4 400G QSFP112 SR4 PAM4 850nm 100m MTP/MPO-12 OM3 FEC Optical Transceiver Module $990.00
-
OSFP-400G-DR4-FLT 400G OSFP DR4 Flat Top PAM4 1310nm MTP/MPO-12 500m SMF FEC Optical Transceiver Module $800.00
-
OSFP-400G-SR4-FLT 400G OSFP SR4 Flat Top PAM4 850nm 30m on OM3/50m on OM4 MTP/MPO-12 Multimode FEC Optical Transceiver Module $650.00
-
OSFP-400G-DR4 400G OSFP DR4 PAM4 1310nm MTP/MPO-12 500m SMF FEC Optical Transceiver Module $900.00
-
OSFP-400G-SR8 400G SR8 OSFP PAM4 850nm MTP/MPO-16 100m OM3 MMF FEC Optical Transceiver Module $480.00