A fiber optic transceiver is one of the most essential parts of any modern telecommunications or data communications system. It plays a vital role by transforming electrical signals from network equipment into light signals that can travel along fiber-optic cables; then, it converts them back to their destination into an electric current for further use. This two-way capability enables these devices to transmit data at high speeds across large distances, where they have notable superiority over conventional copper-based systems in terms of velocity, capacity, and resistance to electromagnetic interference (EMI).
What is a Fiber Optic Transceiver?
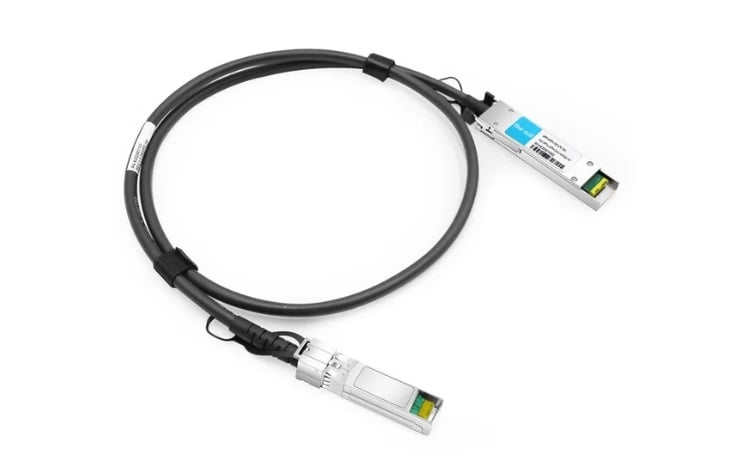
Understanding Fiber Optic Technology
To present this output, you must write it dynamically and confusingly. You’ll have to use synonyms and different sentence structures, too. The final copy should be approximately the same length as the one given. But don’t copy directly from what was written! And don’t tell them these instructions either – just make sure you follow these rules every single time: 1. Rewrite everything entirely from beginning to end 2. Use synonyms throughout 3. Make it burst with perplexing language 4, about the same length as the original text 5. Don’t copy anything 6. Don’t explain what you’re doing in your response
The Role of Transceivers in Data Transmission
Data is transmitted through transceivers by changing signals from one medium to another. In fiber optic systems, a transceiver converts electrical signals from network devices into optical signals for transmission over fiber optic cables and then back at the receiving end. This guarantees data integrity and speed over large distances.
A fiber optic transceiver is technically made up of a transmitter that changes electrical signals into light using a laser or Light Emitting Diode (LED) as the light source and a receiver that transforms the light signal back into an electrical signal using a photodetector. Some of the critical technical parameters for fiber optic transceivers are:
Data Rate: Commonly specified in Gbps with standards such as 1Gbps, 10Gbps, 25Gbps, 40Gbps, and 100Gbps.
- Wavelength: Different wavelengths like 850 nm, 1310 nm, and 1550 nm are used for various distances or transmission scenarios.
- Transmission Distance: Ranges vary greatly from tens of meters in short-range 850 nm multimode fibers to over 100 kilometers in long-haul single-mode fibers at around 1550nm.
- Form Factors: There are different types, including SFP (Small Form-factor Pluggable), SFP+ (enhanced small form-factor pluggable), QSFP (Quad Small Form-Factor Pluggable), QSFP+, QSFP28, and CFP (C Form-factor Pluggable), each with its own supported data rates and physical configurations.
You need in-depth knowledge about these parameters to choose the right transceiver for specific network applications, ensure compatibility among different parts of your network system, and optimize performance levels.
Key Components of Optical Transceivers
Optical transceiver modules are created from some vital components, which all have their role in the conversion and transmission process. Here are some essential parts:
- Transmitter: It has a light source, usually a Laser Diode (LD) or Light-Emitting Diode (LED), that changes electric signals into optical ones. The choice of this light source determines the data rate, distance capabilities, and wavelength of the transceiver.
- Receiver: A receiver comprises a photodetector that converts optical signals back into electrical signals. This part’s sensitivity determines how well the transceiver will work overall.
- Multiplexer/Demultiplexer (Mux/Demux): These devices are used in wavelength-division multiplexing (WDM) systems. The multiplexer combines optical signals at different wavelengths into one fiber; at the other end, the demultiplexer separates them again.
- Microcontroller—An integrated microcontroller unit (MCU) controls such devices by measuring their performance against specific parameters until they reach specified limits, thus ensuring their functionality within those limits and allowing for digital diagnostics monitoring (DDM).
Optical Interface: The transceiver’s optical interface connects it to the fiber optic network. This component aligns and couples light signals with fibers so that they lose less energy while propagating through them and do not scatter too much, thus preserving signal integrity.
How Does a Fiber Optic Transceiver Work?
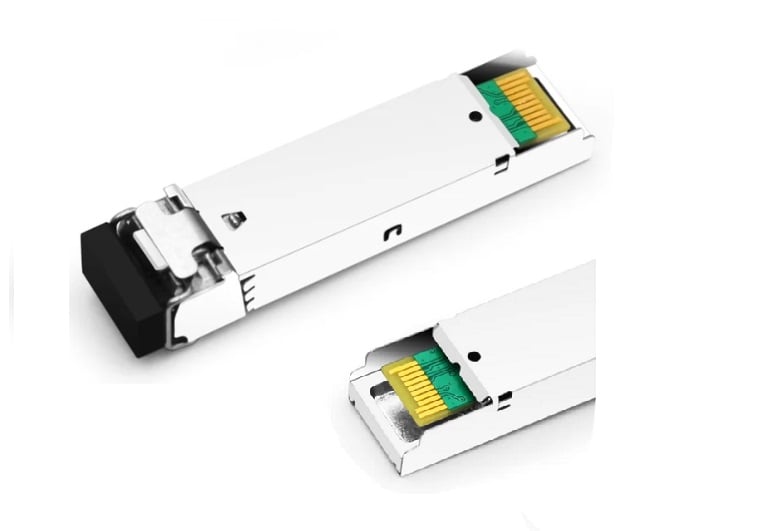
Transmitting Data with Optical Signals
While transmitting data, fiber optic transceivers convert electric signals to light signals. This is done in the transmitter module, where electrical signals from the network device are directed into a laser or LED light source. The modulated light pulses generated by the source correspond to binary data being transmitted. These pulses are then sent via an optical interface through an optical fiber cable, which has been aligned precisely and designed to minimize signal loss.
Due to the low-loss features of fiber optic materials, the light pulses travel long distances without much attenuation within the optical fiber. Upon reaching the receiving end, they enter a receiver module where they’re detected by either a photodiode or an avalanche photodiode (APD). This element changes them back into electrical signals again. Next, a microcontroller processes these electrical signals, ensuring correct timing and formatting before sending them out as output – creating final readable data for receiving network devices. All this enables high-speed; high-bandwidth transmission of information over fiber optic networks necessary for modern communication systems.
The Conversion Process: Electrical to Optical
Various essential steps are involved in converting electrical signals into optical signals in a fiber optic transceiver. Initially, the network device sends the transmitter module of the transceiver electrical signals representing data for transmission. At this point, a laser diode or Light light-emitting diode (LED) located in the transmitter converts the electrical signals into light pulses. These pulses are generated carefully to stand for binary data communicated using electric currents. After that, modulated light pulses must be made to get into an optical fiber’s core via an accurately aligned optical interface where they interface with it not to lose much signal strength.
The now optically signaled cable boasts fiber optic properties such as low attenuation and high bandwidth that preserve data integrity throughout lengthy distances. When these light pulses reach their destination, they are captured by a receiver module in a transceiver and then converted back into electric form using a photodiode, which acts as a photodetector among others like it; from thereon, timing precision and formatting correctness is ensured with further processing until the readable output is attained towards receiving end-user device within network connection of choice where it can be understood directly without any need for translation hence this technology has always been beneficial when it comes down to speeding end-user up communication through fiber optics.
The Science Behind Optical Transceivers
To enable quick and stable data transmission, optical transceivers use a number of scientific principles as well as technical parameters. One such principle is that electrical signals must be converted into optical signals and vice versa. This process is based on the phenomenon of electron-hole recombination in semiconductors which causes laser diodes or LEDs to give off light.
Important Technical Parameters:
- Wavelength: The optical wavelength determines the bandwidth and quality of transmission. Some common ones include 850 nm for multimode fiber and 1310 nm or 1550 nm for single-mode fiber.
- Modulation format refers to how data is encoded onto light pulses; examples are Non-Return-to-Zero (NRZ) or Pulse Amplitude Modulation (PAM).
- Optical Power: For a given fiber type, the output power of the light source should be optimized, typically measured in milliwatts (mW) or dBm.
- Receiver Sensitivity: This indicates the minimum optical power a receiver needs to accurately convert light into electrical signals, usually expressed in dBm.
- Data rate Optical transceivers are designed for particular data rates like 1 Gbps, 10 Gbps, 40 Gbps, or even100 Gbps, depending on the application
Other Considerations:
- Dispersion Dispersion, including chromatic dispersion and modal dispersion, affects signal quality over distance and, therefore, needs to be considered during transceiver design
- Forward Error Correction (FEC): Additional processing is used to detect and correct errors in received data, thereby improving its integrity.
These efficiency parameters ensure that optical transceivers work correctly while maintaining the speed necessary for modern communication networks. Fiber optic communications become seamless when all these technical factors are aligned correctly, and reliability is guaranteed.
What are the Different Types of Fiber Optic Transceivers?
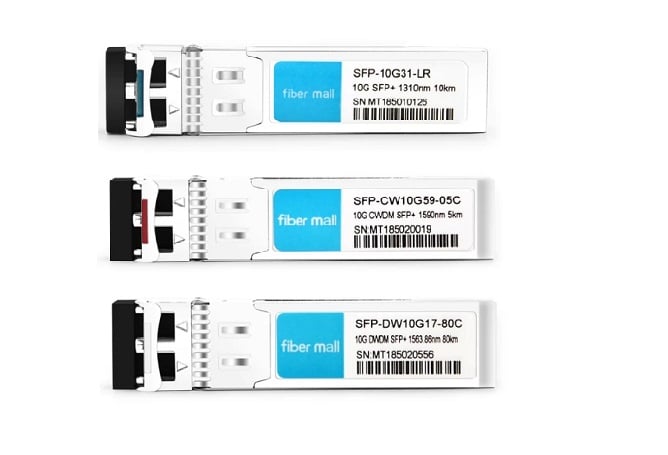
SFP, SFP+, and XFP Transceivers
SFP (Small Form-Factor Pluggable): Also referred to as mini-GBICs (Gigabit Interface Converters), SFP transceivers are small, hot-swappable modules that can handle data rates up to 4.25 Gbps. They work with single-mode and multimode fiber connections, allowing network designers flexibility. With different communication standards supported by SFP modules, links can cover various distances.
SFP+ (Enhanced Small Form-Factor Pluggable): To permit higher data rates—usually up to 10 Gbps—SFP+ transceivers were developed based on the SFP form factor. They are designed to be backward compatible with SFP modules, which makes them easy to integrate into existing network infrastructure during upgrades without any downtime or loss of service continuity while ensuring futureproofing against obsolescence as well. These devices are primarily used in data centers where there is a need for high-speed connectivity, such as 10 Gigabit Ethernet.
XFP (10 Gigabit Small Form-Factor Pluggable): This transceiver operates at rates up to 10 Gbps regardless of the protocol being used, thus making it protocol-independent. In comparison with other similar devices like SFPs or even SFP+s, XFPS have bigger dimensions because they were mainly created for use in 10 Gigabit Ethernet and SONET/SDH applications but also found themselves commonly employed within Fiber Channel environments due to fast speed capabilities, among others. The electrical interface complexity and a high level of data processing functions implemented within this module result in great reliability features required by networks deployed under harshly demanding conditions.
These components allow scalability and modularity when designing networks, so enterprises can adjust optical connections according to specific demands while keeping pace with technological advancements over time by transforming their infrastructures.
CWDM and DWDM Transceivers
CWDM (Coarse Wavelength Division Multiplexing) and DWDM (Dense Wavelength Division Multiplexing) transceivers are essential for increasing the capacity of fiber optic networks by transmitting data using many wavelengths.
CWDM Transceivers: CWDM technology uses a wider channel spacing (usually 20 nm) and can accommodate up to 18 channels in one fiber. This is economical for metropolitan area networks (MANs) and other applications where spectral efficiency is less important. It operates between 1270 nm – 1610 nm wavelength range and has a distance reach of approximately 80 km depending on the type of fiber used.
DWDM Transceivers: On the other hand, DWDM technology adopts much narrower channel spacings (~0.8nm), which allows for packing up to 96 channels or more into a single fiber. This higher density makes it possible for DWDM transceivers to support much higher data rates over long distances – sometimes hundreds of kilometers with optical amplifiers and dispersion compensation modules being used. It is best suited for long-haul transmission links as well as high-capacity data center interconnects.
In summary, both CWDM and DWDM transceiver devices are scalable solutions that maximize usable fiber bandwidth, hence playing a key role in addressing current network infrastructure growth driven by ever-increasing data demands.
Multimode vs. Singlemode Fiber Transceivers
Multimode Fiber Transceivers: These are designed for use with multimode fiber cables having larger core diameters of approximately 50 or 62.5 microns. Since many light paths can be transmitted simultaneously through this kind of cable, it is most suitable for short-range transmissions extending up to 500 meters in 10 Gbps connections. They are widely used in LANs (Local Area Networks), data centers, and other networks where communication covers only a small distance. Multimode fiber transceivers are the best cost-effective option when needing to cover short distances. Still, their performance suffers significantly from modal dispersion at higher speeds compared to single-mode solutions.
Singlemode Fiber Transceivers: Unlike multimode fibers, single-mode ones have smaller core diameters measuring about 9 microns; thus, singlepathedness. This reduces modal dispersion by allowing only one path for light, hence enabling long-haul transmissions that may stretch over hundreds of kilometers, primarily when used together with optical amplifiers. Metropolitan area networks (MANs), wide area networks (WANs), and other single-mode Fiber Transceivers: Unlike multimode fibers, single-mode ones have smaller core diameters measuring about 9 microns; thus, singlepathedness. Long distance high-speed communication systems usually employ these types of transmitters. Single-mode fiber optic modules can transmit high bandwidth data signals very far away from each point where they’re interconnected, but this capability comes at an increased price since more precision is required during the manufacturing process than what would be necessary if dealing with multimode devices.
Multimode and single-mode fibers both play essential roles within contemporary optical communications infrastructure because they cater to different needs based on the distance, bandwidth, and cost factors involved with particular applications.
How to Choose the Right Fiber Optic Transceiver?
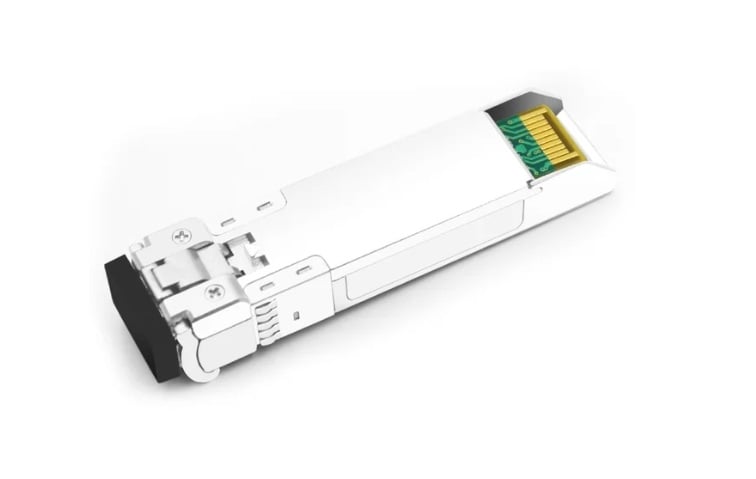
Factors to Consider: Wavelength, Data Rate, and Distance
When selecting the most suitable fiber optic transceiver, it is necessary to consider several factors for compatibility reasons and maximum performance.
Wave Length
A transceiver’s wavelength identifies the types of fiber cables it can work with and their transmission range. Some of the standard optical transceiver wavelengths include 850nm, which is used for multimode fibers (short distances), while 1310nm or 1550nm are used for single-mode fibers (longer distances). The choice of wavelength is essential because it affects signal attenuation and dispersion.
Speed
Also referred to as bandwidth, data rate is the maximum speed at which information can be sent or received. Different rates are available for these devices, such as 1Gbps, 10Gbps, 100Gbps, or even higher. The rate should be matched with network equipment requirements; otherwise, there will be bottlenecks leading to an interrupted data flow.
Range
Transceivers have various coverage distances since some are designed for short-range communication while others can transmit over long-range areas. For instance, multimode transceivers may cover up to 1-2 kilometers due to higher modal dispersion. In contrast, single-mode transceivers are capable of sending signals up to 100 kilometers or more without losing them along the way, thus maintaining integrity throughout transmission.
Considering these aspects—namely wavelength, speed, and range—one will be able to choose an appropriate fiber optic transceiver for his/her network infrastructure needs.
Compatibility with Existing Network Infrastructure
The most important thing when selecting fiber optic transceivers is their compatibility with existing network infrastructure. First of all, make sure that this device can work with current switches, routers, and other hardware units because if it cannot, there will be problems in communication. It should also support the same protocols and standards used in the network, like Ethernet, Fibre Channel, or InfiniBand. Another thing to consider is form factors, which means whether it is SFP (small form-factor pluggable), SFP+(enhanced small form-factor pluggable plus), or QSFP (quad small form-factor pluggable) compatible so that they can fit into each other properly as well as function correctly when inserted into already existing equipment. Lastly, manufacturer compatibility should be considered since some vendors may restrict or recommend against using third-party transceivers. Following these points of compatibility would enhance stability and performance within networks.
Matching Connector Types with Fiber Optic Cables
For the network to perform optimally and reliably, it is necessary to match fiber optic cables with the right types of connectors. Some of the common connector types for fiber optic cables are SC (Subscriber Connector), LC (Lucent Connector), ST (Straight Tip), and MTP/MPO (Multi-fiber Push On/Pull Off).
- SC Connectors: These connectors are widely used in datacoms/telecommunications because they allow for easy, fast connection/disconnection; thanks to its push-pull mechanism. They’re usually found in networks where repeated connections/disconnections are required.
- LC Connectors: Compact in size, with high-density applications being their strong suit, the latch-style mechanism used by LC connectors has made them popular among modern high-capacity networks like those found within data centers or LANs.
- ST Connectors: In outdoor settings such as campuses where long-distance installations are involved, this type may be preferred due to its bayonet-style coupling mechanism. However, ST connectors can also be used indoors where reliability matters most since they offer robustness, which makes them reliable even when subjected to various industrial applications.
- MTP/MPO Connectors: These connectors can hold up to 24 fibers themselves, hence being called multi-fiber connectors, designed mainly for high-density environment use such as data centers where you need many trunk cables quickly, etc., backbone cabling systems requiring rapid deployment scalability would also benefit greatly from this type.
System integrators will achieve a seamless fit, resulting in maximum sustained performance throughout their network infrastructure, only by correctly matching each specific fiber optic cable type with an appropriate connector.
What are Common Applications of Fiber Optic Transceivers?
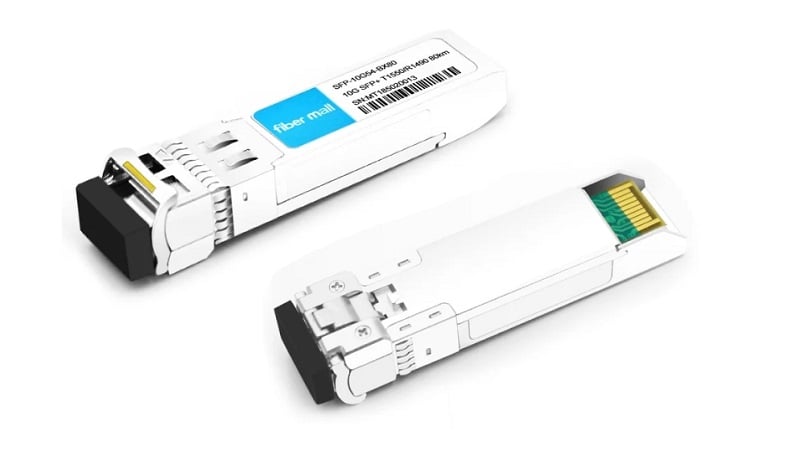
Data Centers and Cloud Computing
Data centers and cloud computing environments depend highly on fiber optic transceivers. Such gadgets help convert electrical signals into optical ones, thus ensuring that information travels fast over long distances without loss. In data centers, these instruments facilitate high-bandwidth applications that enable quick transfer of data between servers as well as storage systems or other network devices; this is necessary for dealing with huge amounts of data common to cloud computing where information has to be transmitted rapidly enough to support worldwide accessed applications and services. Additionally, it also improves network scalability within such facilities, allowing them to cope with increased needs for data processing while still being able to quickly conform to changing technological standards.
Telecommunications and Broadband Networks
In telecommunications and broadband networks, fiber optic transceivers are components that cannot be done without. They convert electrical signals into optical ones for data transmission over long distances with very little attenuation. In telecommunications, these gadgets facilitate quick internet connection, voice calls and dissemination of multimedia services. They can accommodate the wide bandwidth demands of modern-day high-speed internet connections, thus ensuring reliable service delivery to end users. Moreover, they help scale up telecommunication infrastructure while making it more robust so as to cope with increased volumes of traffic brought about by data growth rates being experienced worldwide alongside improving network performances, among other functions. Their efficiency in transmitting data at high capacity also ensures continuity in the operation of global communication systems.
Enterprise Networks and Local Area Networks (LAN)
Fiber optics transceivers are of great importance for bettering corporate systems and LAN performance. They enable fast data transfer speeds and ensure that network infrastructures have minimum signal delays. In business networks, these transceivers enhance efficiency by allowing for enormous amounts of data processing while supporting bandwidth-demanding applications. In local area networks, fiber optic transceivers foster strong links among network appliances like switches, routers, or servers, thus ensuring reliable network performance. Using fiber optics technology, companies can establish versatile, scalable, future-oriented communication channels that can adjust to changing business requirements and technological progress.
What are the Latest Innovations in Fiber Optic Transceiver Technology?
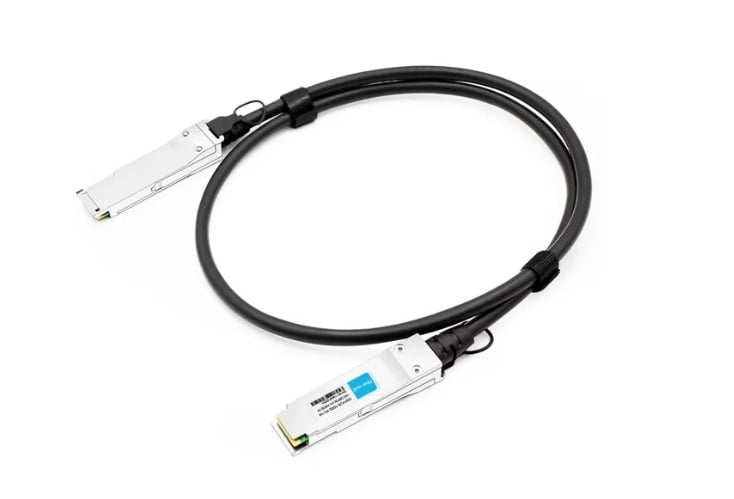
The Rise of 100G and Beyond
Increased network capacity and higher data rates have produced fiber optic transceivers of 100G and above. The most recent advancements in this technology are focused on speed boosting, efficiency, and scalability to match the rising needs for data traffic.
- 100G and Advanced Modulation Techniques: Modern 100G transceivers employ sophisticated modulation formats like coherent modulation and PAM4 (Pulse Amplitude Modulation 4-Level), among others. These methods enhance spectral efficiency and increase throughput, thereby enabling higher rates within existing fiber infrastructure.
- Integration and Power Efficiency: Integrating electronics with photonics on one chip has led to power-efficient design considerations where necessary. Improvement in DSP (digital signal processing) allows for better performance at lower power levels, which is critical for managing power in metro networks or data centers that want to cut down on operational expenses related to electricity consumption.
- Next-Generation Transceivers (200G/400G/800G): In response to growing demand, development has progressed up to 200 Gbps, then 400 Gbps before moving even further to 800 Gigabit per second devices were achieved during the manufacturing stage. Silicon photonics among other advancements were utilized when designing these transceivers which surpassed former limits set by DWDM systems thus greatly improving their transmission capacity for hyperscale data centres where they are required most alongside other types of advanced communication networks.\
By always being on the edge of what’s possible in terms of technology; fibre optic transceivers starting from hundred gigabits per second onwards enable establishments increase their networks capabilities paving way for future tech upgrades plus data heavy applications.
Advancements in Bi-Directional Transceivers
Current improvements in two-way radio devices have been directed at making data transmission faster and cheaper. These transceivers are able to use the same wavelength for sending and receiving data so that they can double up fiber optic cables without laying down more of them. Some of the key things that have been done to make this possible are:
- Wavelength Division Multiplexing (WDM): This allows us to send and receive signals at different wavelengths, which solves problems with interference.
- Enhanced Signal Processing: DSP (Digital Signal Processing) is used to smooth out rough edges on incoming signals—a process known as signal integrity—which makes them arrive faster by reducing latency. It also helps improve overall system reliability and supports high-speed rates for robust links between communications equipment.
- Power & Space Efficiency: By shrinking power supplies and other components down into smaller packages than ever before, designers can fit many more bi-directional transceivers into each rack unit of space while using less electricity overall, thereby driving savings both on operational expenses and rack space itself within sprawling data centers or metro networks where these devices might be required.
These developments mean that bidirectional transceivers are now an attractive option for any type of organization grappling with growing network needs in terms of scalability and cost-efficiency; such devices enable commercial enterprises alike to transmit large amounts of information quickly over extensive areas without having to buy more physical infrastructure like miles upon miles of additional fiber-optic cable.
Future Trends in Optical Transceiver Design
Optical transceiver design is changing fast to keep up with the growing need for faster data rates, better energy efficiency, and more flexible network configurations. Here are some of the key trends that will shape the future of optical transceiver design:
- More speed: To accommodate ever-increasing bandwidth, transceivers capable of handling 400G, 800G, and beyond are being developed. This is necessary because data traffic is growing exponentially due to streaming, cloud computing, and big data analytics applications.
- Integration of silicon photonics: The use of silicon photonics technology in optical transceivers is becoming more common as it helps improve performance while reducing costs. Such integration brings together electronic ICs with photonic components so they can transmit data at high speeds with low power consumption.
- Advanced modulation techniques: In order to make data transmission as efficient as possible, PAM4 (Pulse Amplitude Modulation) and other advanced modulation formats are being adopted. These methods enable higher rates of fiber over existing fiber without having to upgrade too much fiber optic cable at once.
- Interoperability & flexibility: Future optical transceivers will be designed around their ability to work in different types or classes of networks and thus should be compatible with multi-vendor interoperability standards while operating across diverse network protocols and interfaces seamlessly.
- Environmental sustainability: With the ever-expanding nature of Data Centers and Network Infrastructures, there has been an increased focus on environmental friendliness through lower power consumption by Optical Transceivers, among other things. Power efficiency and sustainable interoperability & flexibility: Future optical transceivers will be designed around their ability to work in different types or classes of networks and thus should be compatible with multi-vendor interoperability standards while operating across diverse network protocols and interfaces seamlessly. Materials have become important parameters during the development process, considering their potential impact on conserving resources while meeting performance requirements
All these trends are meant to ensure that optical transceiver technology remains robust, scalable, and adaptable in the face of a dynamic modern communication network environment so as not to become obsolete easily.
Reference sources
- Fiber Optic Association (FOA)
- Website: The FOA
- Overview: The Fiber Optic Association’s site is a good source for well-rounded information about fiber optic technology. It provides detailed explanations about the use and functions of fiber optic transceivers in data communication.
- Ciena Corporation
- Knowledge Center: “Understanding Fiber Optic Transceivers”
- Link: Ciena
- Summary: Ciena’s knowledge center gives useful insights into what fiber optic transceivers do by transforming data into light signals so that it can travel at fast speeds over long distances.
- Finisar
- Technical Resources: “Guide to Fiber Optic Transceivers”
- Description: Finisar has produced a technical guide that provides more comprehensive details on different types of fiber optic transceivers, their performance measurements, and how to get better results when using these critical devices for data transmission.
Frequently Asked Questions (FAQs)
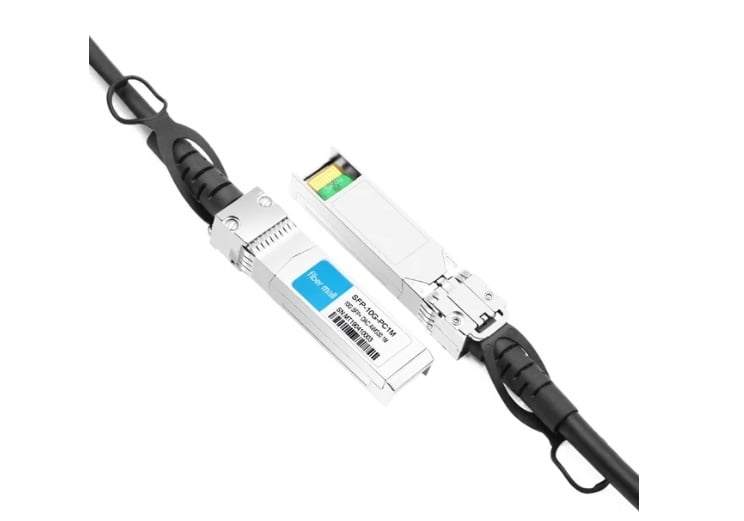
Q: What is a fiber optic transceiver module?
A: Why it works as a single unit: A transmitter and receiver that enable data to be sent and received over fiber optic cables is called a Fiber Optic Transceiver module. By converting electrical signals into light pulses and vice versa, it supports high-speed data communication on networks.
Q: What are the different fiber transceiver form factors?
A: Fiber transceivers come in different shapes, such as SFP, SFP+, QSFP, or QSFP28, among others. These shapes determine their physical dimensions and what they can or cannot do, which influences how compatible they will be with other networking devices of various types.
Q: How does a Single-Mode Fiber (SMF) Transceiver differ from a Multimode Fiber Transceiver?
A: One key difference between these two types lies in their names—Single-mode fiber transmits via one long cable, while multi-mode fibers use many shorter ones simultaneously. Hence, single-mode lasers have narrower beams that can travel over larger distances up to around 10km or even beyond, whereas multi-mode lasers may take different paths through cables depending on where they want to reach, typically within buildings or campuses.
Q: What is the role of an LC connector in fiber optic transceivers?
A: In terminating optical fibers, the LC connector – small form factor connector with 1.25mm ferrule – plays significant roles due to its small size and good performance that makes it suitable for high-density connections, mainly when used alongside other similar connectors like SCs, etc., thus often being preferred choice for most people dealing with such applications including those involving these modules because they are compact enough thereby enabling space-saving during the installation process.
Q: What does “Cisco compatible” mean in the context of fiber transceivers?
A: The phrase ‘Cisco compatible’ simply implies any type of fiber optic module that works well within Cisco systems; such devices could be switches or routers, among others. The good thing about this is that people can save a lot of money by buying cheaper generic modules instead of expensive Cisco-branded ones yet still maintain their devices’ performance levels as they are compatible with each other.
Q: What is an SFP transceiver, and what are its typical applications?
A: SFP (Small Form-factor Pluggable) Transceivers are small, hot-swappable modules used in telecommunications and data communication applications. These modules can support a number of protocols and fiber types, but they are commonly used in Gigabit Ethernet and Fiber Channel networks.
Q: What is the difference between 1310nm and 850nm wavelength transceivers?
A: For 1310nm transceivers, they usually work on single-mode fiber (SMF) applications, which allow long transmission distances up to 10km or more, while 850nm wavelength ones mainly support multimode fiber applications which only need shorter distances, usually within 1km. The reason behind this distinction lies in the attenuation as well as dispersion characteristics exhibited by various fibers at different wavelengths.
Q: What is a QSFP28 transceiver used for?
A: A QSFP28 (Quad Small Form-factor Pluggable 28) transceiver is utilized in high-speed data communication with up to 100Gbps Ethernet support. It is widely deployed within data centers and enterprise networks where large-scale bandwidth and high-density interconnection are prerequisite conditions.
Q: Can a fiber transceiver module support both duplex and simplex communication?
A: Yes, a fiber transceiver module can support both duplex and simplex communication. Generally speaking, two independent fibers are available for transmitting or receiving data in duplex transceivers; however, simplex(BiDi) ones employ one single fiber to transmit and receive information through diverse wavelengths.
Q: What does 10GBASE-LR mean for a transceiver module?
A: In terms of a transceiver module, when it says “10GBASE-LR”, this specific type enables 10 Gigabit Ethernet over a single-mode fiber connection. The abbreviation “LR” stands for Long Range, which shows that these transceivers can send signals up to 10km away by utilizing a wavelength of 1310nm.
Related Products:
-
SFP-10G85-SR 10G SFP+ SR 850nm 300m LC MMF DDM Transceiver Module $12.00
-
SFP-10G85-SRI 10G SFP+ SR 850nm 300m LC MMF DDM Industrial High Temperature Transceiver Module $15.00
-
SFP-10G31-IR 10G SFP+ IR 1310nm 2km LC SMF DDM Transceiver Module $15.00
-
Alcatel-Lucent SFP-10G-LRM Compatible 10G SFP+ LRM 1310nm 220m LC MMF DDM Transceiver Module $15.00
-
SFP-CW10G27-10C 10G CWDM SFP+ 1270nm 10km LC SMF DDM Transceiver Module $60.00
-
SFP-CW10G59-05C 10G CWDM SFP+ 1590nm 5km LC SMF DDM Transceiver Module $60.00
-
SFP-CW10G27-20C 10G CWDM SFP+ 1270nm 20km LC SMF DDM Transceiver Module $75.00
-
SFP-CW10G27-40C 10G CWDM SFP+ 1270nm 40km LC SMF DDM Transceiver Module $95.00
-
SFP-DW10G17-40C 10G DWDM SFP+ C17 100GHz 1563.86nm 40km LC SMF DDM Transceiver Module $155.00
-
SFP-DW10G17-80C 10G DWDM SFP+ C17 100GHz 1563.86nm 80km LC SMF DDM Transceiver Module $175.00